Visual Abstract
Abstract
Cancer remains a formidable global health challenge, necessitating the development of innovative and targeted therapeutic strategies. Photothermal therapy (PTT) has emerged as a promising approach, leveraging light-absorbing agents to selectively destroy cancer cells while sparing healthy tissues. Among these agents, gold nanorods (AuNRs) have garnered significant attention due to their unique optical, thermal, and biocompatible properties. AuNRs exhibit strong absorption in the near-infrared (NIR) region, enabling deep tissue penetration and efficient conversion of light into localized heat, which induces cancer cell ablation. Their surface can be functionalized with targeting ligands, enhancing precision and therapeutic efficacy. However, the cytotoxicity of cetyltrimethylammonium bromide (CTAB), a common surfactant used in AuNR synthesis, poses a challenge. Recent advancements in surface modification and functionalization have addressed this issue, improving biocompatibility and targeting capabilities. This paper explores the unique properties, functionalization, and therapeutic mechanisms of AuNRs in cancer PTT, highlighting their potential in synergistic chemo-photothermal therapy. By integrating AuNRs with chemotherapeutic agents and stimuli-responsive drug delivery systems, researchers have developed innovative platforms that enhance tumor targeting, controlled drug release, and therapeutic outcomes. Case studies demonstrate the efficacy of AuNR-based systems in overcoming drug resistance and improving cancer treatment. This review underscores the transformative potential of AuNRs in revolutionizing cancer therapy, offering a minimally invasive, highly effective, and targeted approach to combat this complex disease.
Cancer, Chemotherapy, Chemo-photothermal therapy, Gold nanorods, Photothermal therapy, Synergistic therapy
INTRODUCTION
Cancer remains one of the most challenging diseases to treat, driving the need for innovative and targeted therapeutic strategies [1]. Among the emerging technologies, Photothermal therapy (PTT) has gained significant attention due to its potential to selectively destroy cancer cells while minimizing damage to healthy tissues. The PTT is an innovative treatment approach that utilizes light energy to generate heat, targeting and destroying tumor cells locally. When the temperature in the tumor microenvironment rises above 41°C, the tumor tissue experiences varying levels of damage. In contrast to conventional tumor treatments like surgery, radiotherapy, and chemotherapy, PTT offers advantages such as fewer side effects, minimal invasiveness, lower equipment costs, and precise targeting capabilities. For PTT to be effective, it requires a laser capable of penetrating healthy tissue to reach the tumor site and a photothermal agent with high efficiency in converting light to heat [2]. AuNRs have emerged as a promising nanomaterial for PTT, owing to their unique optical and physicochemical properties [3]. Gold nanorods (GNRs) stand out due to their exceptional photothermal conversion capabilities. GNRs not only exhibit high chemical stability and ease of modification, but they also offer the advantage of tunable wavelength, setting them apart from traditional gold nanoparticles. These rod-shaped nanoparticles exhibit strong absorption in the NIR region, a wavelength range where biological tissues are relatively transparent [4]. When exposed to NIR light, AuNRs efficiently convert light energy into heat [5], inducing localized hyperthermia that can selectively ablate cancer cells [6]. Additionally, their surface can be functionalized with targeting ligands, enabling precise delivery to tumor sites [7]. This combination of targeted delivery and controlled thermal ablation positions gold nanorods as a powerful tool in the fight against cancer, offering a minimally invasive and highly effective treatment modality [8]. This introduction explores the role of AuNRs in cancer photothermal therapy [9–12], highlighting their synthesis [13], functionalization [14–18], and therapeutic mechanisms [19], as well as their potential to revolutionize cancer treatment [20]. AuNRs have emerged as a promising tool in the field of cancer PTT due to their unique optical [21], thermal [22], and biocompatible properties [23]. PTT is a minimally invasive treatment that uses light-absorbing agents to generate heat and selectively destroy cancer cells [2].
This review summarizes the potential of gold nanorods (AuNRs) in revolutionizing cancer therapy, particularly through PTT and synergistic chemo-photothermal approaches. In addition, we will review the properties of AuNRs and their recent advancements in surface modification and functionalization strategies.
UNIQUE PROPERTIES OF GOLD NANORODS
Gold nanorods exhibit strong absorption in the NIR region (700–1100 nm) due to their longitudinal surface plasmon resonance [24]. This is advantageous because NIR light can penetrate deeper into biological tissues with minimal scattering and absorption, allowing for targeted treatment of tumors located beneath the skin [25]. Among the various metal nanoparticles, AuNRs have garnered significant attention due to their unique anisotropic shape [26], which gives rise to tunable optical properties [27] and distinct surface plasmon resonance (SPR) modes [28]. The SPR is a fascinating optical phenomenon that occurs when free electrons at the surface of a metal nanostructure collectively oscillate in response to incident light [29]. This oscillation, known as a surface plasmon, enables precise control over light-matter interactions, making them valuable in biomedical and optoelectronic applications [30], and is highly sensitive to the size [31], shape [32], composition [33], and surrounding environment of the nanostructure [34]. The Au nanorods exhibit two primary SPR modes: a transverse mode, corresponding to electron oscillations along the short axis of the rod that is insensitive to the changes in the size of the AuNRs and the surrounding refractive index [35], and a longitudinal mode, associated with oscillations along the long axis that shows a red shift with the increase of the aspect ratio of AuNRs and is very sensitive to any change in the refractive index (Figure 1) [36]. The longitudinal plasmon band is the key factor in AuNR performance for PTT because it determines optimal laser wavelength selection (NIR for deep tissue), photothermal conversion efficiency, and stability under laser irradiation. The longitudinal mode is particularly interesting because it can be tuned across a wide range of the electromagnetic spectrum, from visible to NIR wavelengths [37], by simply adjusting the aspect ratio (length-to-width ratio) of the nanorods [38]. The AuNRs, with various aspect ratios and suspended in aqueous solutions, display a range of different colors (Figure 2) [36]. This tunability makes gold nanorods highly versatile for applications in sensing [15, 39–43], imaging [44–47], photothermal therapy [48–51], and catalysis [52–56]. The color of AuNR solutions corresponds to their LSPR peaks, making them useful for biomedical imaging and therapy. Short AuNRs (lower aspect ratio, ~2–3) with red color and localized surface plasmon resonance (LSPR) peak ~520–600 nm (transverse plasmon mode) are less effective for deep-tissue imaging but useful for surface-enhanced Raman spectroscopy (SERS) and superficial imaging. Medium AuNRs pose a greenish-blue color (aspect ratio ~3–4) and longitudinal LSPR ~650–800 nm. They are suitable for shallow tissue imaging and photothermal therapy (PTT) due to moderate near-infrared (NIR) absorption. Long AuNRs have a dark blue/purple color (higher aspect ratio ~4–5+) and longitudinal LSPR ~800–1100 nm, extending into NIR-II. They are ideal for deep-tissue imaging and therapy because of strong NIR absorption, which minimizes tissue scattering and autofluorescence.
The strong localized electric fields generated at the surface of gold nanorods during SPR enhance their interaction with light and nearby molecules [57], making them excellent candidates for surface-enhanced spectroscopy [27] and biosensing [58]. Additionally, the ability to absorb and convert light into heat has positioned gold nanorods as effective agents in photothermal applications, such as cancer therapy [10]. Understanding the principles of SPR in gold nanorods is crucial for optimizing their design and harnessing their potential in nanotechnology and biomedical applications [59]. This introduction explores the fundamental aspects of SPR in gold nanorods, their unique optical properties, and their growing role in advancing science and technology [60]. Gold nanorods efficiently convert absorbed NIR light into heat, making them ideal for inducing localized hyperthermia in cancer cells [61].
SURFACE MODIFICATION OF THE CTAB-CAPPED AuNRs
The toxicity of AuNRs is primarily attributed to the surfactant CTAB, which presents a major obstacle to the clinical development of AuNRs-based PTT [62]. Surface modification of AuNRs improves their stability, such as the replacement of weakly bound CTAB with more stable ligands [63], biocompatibility, such as silica coating [64], and targeting efficiency [65], thereby boosting their therapeutic potential. These modified AuNRs can circulate throughout the body, accumulate at specific target sites, enhance cellular uptake, prolong blood circulation, and improve both biocompatibility and targeted delivery to diseased tissues [66]. Their key role in PTT arises from their capacity to absorb and convert NIR light into heat [23], selectively destroying cancerous tissues while sparing surrounding healthy cells because NIR light causes minimal harm to non-targeted regions due to low absorption by biological tissues [67]. Additionally, AuNRs can be incorporated into stimulus-responsive drug delivery systems, where NIR-induced heating enables controlled drug release [68]. Various functionalization approaches have been investigated to customize AuNRs for specific biomedical applications [15, 69–71].
Yu et al. developed an innovative nanoparticle-based strategy using MUC18-targeted gold nanorods combined with mild hyperthermia to specifically enhance tumor endothelial permeability. This approach improves the efficacy of traditional cancer therapies, such as photothermal therapy and anticancer drug delivery, by facilitating the transport of photo-absorbers and drugs across the tumor endothelium. Using single-cell imaging and molecular biology techniques, the study demonstrates that MUC18-targeted gold nanorods and mild hyperthermia enlarge intercellular gaps in tumor endothelium by inducing circumferential actin remodeling, stress fiber formation, and contraction of adjacent endothelial cells. Given that MUC18 is overexpressed in various tumor endothelial and cancer cells, this method offers a promising strategy to actively enhance tumor endothelial permeability and improve cancer therapy outcomes. Building on these findings, the study introduces MUC18-targeted gold nanorods combined with mild hyperthermia induced by near-infrared laser irradiation to specifically boost tumor endothelial permeability and enhance the efficacy of photothermal therapy and drug delivery (Figure 1). The MUC18-targeted gold nanorods are designed to serve multiple functions: (a) targeting tumor endothelial cells, (b) disrupting MUC18 downstream signaling to enhance endothelial cell contraction and the EPR effect, and (c) targeting MUC18-positive cancer cells to improve photothermal ablation efficacy. Overall, this approach represents a significant advancement in cancer therapy by actively and specifically inducing the EPR effect in tumor endothelium, thereby enhancing the effectiveness of treatments such as chemotherapy and photothermal therapy (Figure 3) [72].
Foti et al. developed a straightforward method for the effective removal of CTAB through a combination of ligand replacement and surface bioconjugation. This process not only eliminates CTAB but also functionalizes the nanorods with hyaluronic acid (HA), enhancing biocompatibility and introducing cancer cell-targeting capabilities. The photothermal response of the nanoparticles was evaluated through laser irradiation and thermal camera measurements. In vitro proof-of-concept studies were conducted on prostate cancer cells (PC–3), which overexpress the CD44 receptor targeted by HA, and compared with CD44-negative murine fibroblasts (3T3 cell line). Cytotoxicity and oxidative stress were assessed using MTT and MitoSOX assays, respectively. Cellular uptake, organelle alterations, and effects on cell migration were examined using confocal laser scanning microscopy (LSM) and optical microscopy (wound scratch assay). The results demonstrated the potential of this approach to reduce cytotoxicity, enhance targeted therapeutic efficacy, and control scar tissue formation, thereby optimizing gold nanorods for cancer treatment. This study highlights the promising application of CTAB-free, HA-functionalized AuNRs in nanomedicine, particularly for targeted cancer therapy, by improving biocompatibility and photothermal performance [73]. Gold nanorods are pivotal in applications such as plasmonic imaging [74], small molecule detection [75], and photothermal therapy [76]. However, their full potential is hindered by challenges in DNA functionalization [70]. To address this, Chen et al. developed a convenient method for the rapid assembly of polyadenine (polyA)-tailed DNA on AuNRs, allowing control over DNA density, rigidity, and valence. They investigated the impact of these designed DNA properties on the construction of core−satellite structures, using AuNRs as cores and spherical gold nanoparticles (AuNSs) as satellites. Their findings highlight that DNA density, rigidity, and valence are critical factors for efficient assembly. PolyA-tailed DNA was shown to modulate DNA density, reduce spatial hindrance, and minimize electrostatic repulsion, thereby facilitating the construction process. Additionally, enhancing DNA rigidity (e.g., transitioning from single-stranded to double-stranded) and incorporating multiple binding sites further improved assembly efficiency. In this study, the rapid and efficient assembly of polyA-tailed DNA on AuNRs was achieved using a butanol dehydration method. It was determined that a minimum polyA length of 50 nucleotides is required for successful assembly, and the DNA density could be controlled by adjusting the polyA length. The study also explored the effects of thiolated and polyA-tailed DNA, with varying lengths and structures (single- or double-stranded), on the assembly of core−satellite structures.
By manipulating the spatial distribution of DNA bonds on AuNR surfaces, key factors such as density, rigidity, and valence were identified as essential for optimizing assembly efficiency. Specifically, polyA-tailed DNA reduced spatial hindrance and electrostatic repulsion at DNA binding sites, enhancing the assembly of core−satellite structures. Furthermore, increasing DNA rigidity and incorporating multiple binding sites contributed to even greater efficiency in the assembly process (Figure 4) [70].
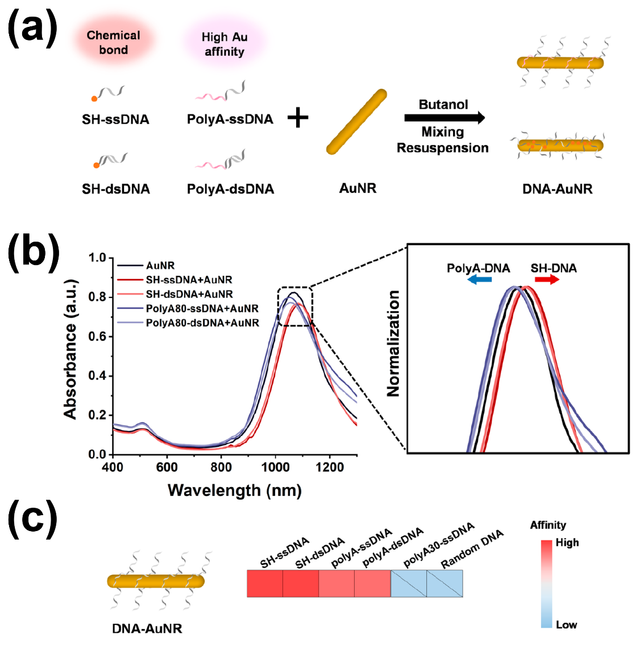
Over the past few decades, NIR-controlled drug delivery systems have garnered significant attention due to their deep tissue penetration and minimal side effects [77]. Reis et al. developed an innovative approach for gastric cancer treatment by integrating photothermal infrared-sensitive gold nanorods with a conjugated microporous polymer (CMP) to create a targeted drug delivery system for the cytostatic drug 5-fluorouracil (5-FU). CMPs, known for their fully conjugated networks and high internal surface areas, can be precisely tailored for the adsorption and transport of active compounds through specific chemical functionalities. By incorporating surfactant-stabilized AuNRs into the CMP synthesis in dimethylformamide (DMF), the surfactant shell is destabilized and replaced by the CMP. Notably, low initial surfactant concentrations result in a uniform distribution of AuNRs within the polymer matrix, while the AuNRs retain their plasmonic properties, as confirmed by electron energy loss spectroscopy. This ensures the hybrid material exhibits significant photothermal properties, demonstrated in proof-of-principle experiments. In simulated gastric conditions, 5-FU release studies were conducted with and without NIR irradiation. The results revealed that NIR-induced increased Brownian motion not only accelerates drug release but also enhances the total released amount by altering the adsorption-desorption equilibrium. This precise control over the release process highlights the potential of the material for targeted drug delivery. The synthesis involves AuNRs with defined geometric parameters, colloidally stabilized with CTAB in water, which are added to the CMP synthesis (Figure 5) [78].
ADVANCES IN SYNERGISTIC CHEMO-PHOTOTHERMAL THERAPY
Photothermal therapy is a promising strategy for treating malignant tumors [50, 79, 80], particularly when combined with chemotherapy [81–85]. A key component of this approach is the use of photothermal agents capable of efficient photothermal conversion in the NIR-II region [86–91]. Xing et al. developed a novel thermosensitive drug-loaded nanovesicle, USGRV–17–AAG, designed for combined NIR-II photothermal therapy and chemotherapy of solid tumors. These nanovesicles are formed through the self-assembly of gold nanorods modified with amphiphilic polymers (PEG45-b-PS) and UCST-type (upper critical solution temperature) thermosensitive polymers (P(AAm-co-AN)) and are loaded with 17-AAG, an inhibitor of heat shock protein 90 (HSP90). Under 1064 nm laser irradiation, USGRV–17–AAG exhibits a high photothermal conversion efficiency of 65.1 %, enabling the temperature-responsive release of 17-AAG. This dual approach effectively eliminates tumor cells and inhibits HSP90 expression, overcoming tumor heat resistance and enhancing therapeutic outcomes. In vivo studies demonstrated that intravenous injection of USGRV–17–AAG followed by 1064 nm laser irradiation resulted in significant tumor ablation, with a tumor growth inhibition rate of 98.86 %. The surface of the nanovesicle is coated with smart-responsive UCST polymers, allowing precise control over drug release and improving the efficacy of the combined therapy. The gold nanorods used in the nanovesicles have a localized surface plasmon resonance (LSPR) peak in the NIR-II region, maximizing absorption at 1053 nm and enabling efficient photothermal conversion. This innovative system not only achieves outstanding NIR-II photothermal therapy but also demonstrates efficient drug delivery capabilities, offering a promising alternative for anti-tumor treatment. The integration of NIR-II photothermal therapy with chemotherapy via USGRV–17–AAG represents a significant advancement in the treatment of malignant tumors (Figure 6) [92].
Despite significant advancements in cancer treatment, chemotherapy remains a viable option, particularly when combined with photothermal therapy to target resistant breast cancer cells more effectively [14, 93–95]. Okuyucu et al. developed an innovative drug delivery system, (DOX-OA+VERA+AuNRs)@NLC, designed for dual chemo-photothermal therapy against multiple-drug-resistant breast cancer. This system utilizes Type-III nanostructured lipid carriers (NLCs) as a nano-in-nano drug delivery platform, offering enhanced drug loading and controlled release. Doxorubicin (DOX), an antitumor agent, was ion-paired with oleic acid (OA) to increase its loading capacity and reduce burst release, while verapamil (VERA) was co-loaded as a chemosensitizer to counteract drug resistance. Gold nanorods were incorporated as PTT agents, creating a synergistic effect with chemotherapy. The system was further functionalized with a folic acid-chitosan (FA-CS) coating to enable targeted delivery and specific release within cancerous tissues, minimizing damage to surrounding areas. In vitro studies demonstrated the controlled release of DOX-OA and VERA triggered by NIR laser irradiation, ensuring higher concentrations of active pharmaceutical ingredients (APIs) at the target site. The dual drugs and AuNRs were encapsulated within the optimal NLC structure, and cumulative release was evaluated at pH 5.5 and 7.4, aiming to enhance chemotherapeutic efficacy by achieving greater VERA release compared to DOX-OA. The AuNRs, with their optimal size, not only served as effective PTT agents to kill cancer cells but also helped regulate drug release. The system was tested on the MDA–MB–231 triple-negative breast cancer cell line, known for its resistance to treatment, and demonstrated promising results in overcoming drug resistance. These findings highlight the potential of this smart, functionalized, and state-of-the-art drug delivery system (DDS) in treating breast cancer, even in cases of multiple-drug resistance (MDR), by combining chemotherapy and PTT for improved therapeutic outcomes (Figure 7) [96].
Ovarian cancer, a group of disorders originating in the ovaries, fallopian tubes, or peritoneum, is increasingly recognized as resistant to chemotherapy alone [97]. To address this, Fan et al. developed a novel dual chemo/photothermal treatment using nanostructured lipid carriers (NSLCs) loaded with camptothecin (CPT) and gold nanocomposites (AuNCs), termed CPT + AuNCs@NSLCs. These AuNCs, including gold nanorods (AuNRs) and gold nanospheres (AuNSs), were incorporated into NSLCs to leverage their photothermal properties and enhance drug delivery for combined chemo/photothermal therapy. The NSLCs were tested for CPT release before and after exposure to NIR light (808 nm), with AuNRs demonstrating the highest heating potential (ΔT = 22 °C in 5 min at 19.8 µg Au/mg lipids). In vitro studies on HeLa ovarian cancer cells revealed that CPT + AuNRs@NSLCs, under 5 minutes of NIR exposure, achieved the most significant growth inhibition (81%). The photothermal efficiency of AuNCs was found to be structure-dependent, with the cytotoxic effects of CPT and localized hyperthermia from AuNCs synergistically enhancing cellular damage. This study highlights the potential of these advanced nanoformulations as effective chemo/photothermal agents. The research also evaluated the photothermal efficiency of AuNRs and AuNSs under 808 nm NIR irradiation, focusing on targeted CPT release and in vitro cytotoxicity. Results demonstrated that CPT + AuNRs@NSLCs exhibited the highest cytotoxic impact under NIR, with significantly reduced cell viability compared to CPT NSLCs and AuNRs@NSLCs administered separately. Overall, this work showcases the promise of lipid-mediated multifunctional nanoplatforms for combination therapy, emphasizing the synergistic antitumor effects achieved by combining inorganic nanoparticles of optimal shape and concentration with lipid-based drug delivery systems [98]. Nanosystems capable of sequentially delivering multiple drugs with different targets are highly sought after for advancing cancer therapy [99–101]. Current strategies often rely on tumor microenvironment triggers, such as low pH or enzyme activity, for drug release [102–105]. To address this, Costa-e-Sa et al. developed an innovative NIR light-triggered sequential delivery system using lipid-gated mesoporous silica-coated gold nanorods and chitosan/alginate nanogels. The system features mesoporous silica particles (99 ± 11 nm) loaded with methotrexate (MTX) and coated with a thermoresponsive phospholipid bilayer that acts as a gatekeeper. These particles are embedded in a chitosan/alginate nanogel matrix containing DOX. The plasmonic nanogels demonstrated high loading efficiencies of approximately 90% for MTX and 85% for DOX, with an average hydrodynamic diameter of around 300 nm, low polydispersity, and a highly negative zeta potential (~−30 mV). The system enabled sustained drug release under both acidic and neutral conditions, with NIR laser irradiation significantly enhancing the release of both drugs. Notably, DOX exhibited a faster release rate than MTX under acidic conditions. This NIR-triggered sequential delivery strategy holds great promise for delivering diverse drug combinations targeting multiple aspects of the tumor microenvironment, making it a valuable tool in multimodal cancer therapy. The study aimed to develop self-assembled plasmonic nanogels for the sequential release of DOX and MTX via NIR light activation, combining hyperthermia and chemotherapy for enhanced antitumor efficacy. The promising results of the system and straightforward design highlight its potential for delivering other therapeutic drug combinations in cancer treatment and beyond [106]. We developed a novel biocompatible nanocomposite, GNRs@SBA–15-SH, composed of gold nanorods (GNRs) coated with rod-like mesoporous silica SBA–15–SH particles, as a synergistic therapeutic platform for tumor eradication. This system combines photothermal therapy and chemotherapy to deliver heat and drugs, such as DOX, directly to cancer cells. DOX was loaded into the GNRs@SBA–15-SH nanocomposite, and its therapeutic potential was evaluated using A549 human lung cancer cells. The results highlight the high photothermal efficiency of the nanocomposite, thermoresponsive properties, exceptional DOX loading capacity, and biocompatibility. Under 808 nm laser irradiation, the GNRs@SBA–15-SH/DOX nanocomposite demonstrated remarkable synergistic chemo-photothermal effects, significantly enhancing cancer cell ablation compared to individual therapies. The coating of GNRs with mercaptosilane-functionalized SBA–15 nanoparticles not only improved the photothermal activity of GNRs but also increased drug loading capacity. In vitro studies confirmed the biocompatibility and antitumor efficacy of GNRs@SBA–15-SH/DOX against A549 cells. Notably, the synergistic effect of PTT and chemotherapy was attributed to the NIR light-triggered intracellular release of DOX, showcasing the potential of this combined approach for effective cancer treatment [107]. We also designed a novel nanocomposite, gold nanorods conjugated with thiolated β-cyclodextrin loaded with doxorubicin (AuNRs@S-β-CD-DOX), to enhance therapeutic efficacy and mitigate the toxicity of gold nanorods. This nanocomposite exhibits strong absorption in the NIR region, as demonstrated by UV-vis absorption spectra, making it a highly suitable candidate for combined photothermal therapy and chemotherapy. The integration of these therapies is expected to significantly reduce the viability of A549 lung cancer cells in vitro, highlighting its potential as a groundbreaking biomedical material for cancer treatment. The AuNRs@S-β-CD-DOX nanocomposite demonstrates excellent photothermal efficiency, high DOX loading capacity, and remarkable biocompatibility. Thiolated β-cyclodextrin (SH-β-CD) was utilized as a linker to functionalize the gold nanorods, enabling enhanced drug loading through host-guest interactions and facilitating the incorporation of DOX both on the surface and within the nanocomposite. In vitro studies confirmed the biocompatibility and potent anticancer efficacy of AuNRs@S-β-CD-DOX against A549 cancer cells. The results revealed that the synergistic combination of photothermal therapy and chemotherapy significantly enhances cancer cell killing, driven by NIR light-triggered intracellular DOX release. This innovative approach underscores the potential of AuNRs@S-β-CD-DOX as a highly effective platform for cancer therapy (Figure 8) [108].
In this study, we developed β-cyclodextrin-based nanosponges encapsulating PEGylated gold nanorods and DOX, termed AuNR-S-PEG.β-CD NS-DOX. The AuNR-S-PEG.β-CD NS nanocomposite demonstrated high photothermal conversion efficiency and significant drug loading capacity, attributed to the porous structure of the nanosponges. The drug-loaded nanocomposites exhibited pH/NIR dual-responsive drug release, excellent biocompatibility, and efficient uptake by A549 lung cancer cells. Moreover, they showed superior cancer cell-killing efficiency compared to individual chemo- or photothermal therapies. The synthesis process involved creating AuNRs, functionalizing them with thiolated polyethylene glycol (PEG-SH) via Au−S bonds to form AuNR-S-PEG, and encapsulating them into β-cyclodextrin-based nanosponges (AuNR-S-PEG.β-CD NS). DOX was then loaded into the nanocomposite through host−guest interactions. The resulting AuNR-S-PEG.β-CD NS-DOX nanocomposite was thoroughly analyzed for physicochemical properties, drug loading, and release behavior. In vitro studies on A549 lung cancer cells confirmed its pH/NIR dual-responsive drug release and high efficacy in combined chemo-photothermal therapy. Overall, these findings highlight AuNR-S-PEG.β-CD NS-DOX is a promising candidate for advanced lung cancer treatment (Figure 9) [109].
To address the complexities of cancer therapy, this project introduces a novel synergistic approach by combining chemo-photothermal treatment using AuNRs supported on thiol-functionalized mesoporous silica (MCM–41). This innovative strategy offers a promising solution for enhanced lung cancer therapy. The mesoporous MCM–41 was synthesized via a surfactant-templated sol-gel method, selected for its porous structure, biocompatibility, and non-toxic properties. Thiol functionalization of MCM–41 was achieved through a simple grafting process, enabling the synthesis of AuNRs supported on thiol-functionalized MCM–41 (AuNR@S-MCM–41) via gold-thiol interactions. The nanocomposite was further loaded with the anticancer drug DOX, resulting in AuNR@S-MCM–41-DOX. This nanocomposite exhibited pH/NIR dual-responsive drug release behaviors, enabling targeted drug delivery triggered by the tumor microenvironment and NIR laser irradiation. It demonstrated exceptional biocompatibility and efficient internalization into A549 lung cancer cells. Notably, the combined chemo-photothermal therapy using AuNR@S-MCM–41-DOX showed superior efficacy in killing cancer cells compared to individual chemo- or photothermal therapies. The integration of gold nanorods, thiol-functionalized mesoporous silica, and pH/NIR dual-responsive drug release provides a comprehensive and effective therapeutic approach for lung cancer treatment. In this study, gold nanorods were supported on thiolated MCM–41 via Au–S bonds, and DOX was loaded into the nanocomposite through strong electrostatic interactions. Under NIR irradiation, the AuNR@S-MCM–41-DOX nanocomposite demonstrated pH/NIR dual-responsive drug release, enhancing the efficacy of combined chemo-photothermal therapy. In vitro experiments confirmed its biocompatibility and effectiveness against A549 lung cancer cells. The stimuli-responsive drug delivery performance of the nanocomposite, facilitated by internal (tumor microenvironment) and external (NIR laser) triggers, highlights its potential as a promising candidate for advanced lung cancer therapy. Future advancements based on this strategy hold significant promise for addressing cancer challenges and transforming patient care (Figure 10) [110].
CHALLENGES, LIMITATIONS, AND SAFETY CONSIDERATIONS
AuNRs are among the most effective photothermal agents due to strong LSPR in the NIR range (700–1100 nm), optimizing tissue penetration, high photothermal conversion efficiency (~90%), superior stability, and favorable safety when properly coated [111]. The aspect ratio of AuNRs significantly affects their photothermal therapy (PTT) performance by their tunable plasmonic absorption, photothermal conversion efficiency, and cellular uptake and tumor accumulation [112].
Delivery of AuNRs to tumor tissues can be achieved through two primary strategies: passive targeting and active targeting. While both approaches aim to enhance tumor accumulation, they differ significantly in their mechanisms, advantages, and limitations [13].
Passive targeting relies on the enhanced permeability and retention (EPR) effect, a phenomenon where nanoparticles accumulate in tumor tissues due to the leaky vasculature and impaired lymphatic drainage of solid tumors [113]. The mechanism is that disorganized, fenestrated blood vessels in tumors allow nanoparticles (typically 10–200 nm) to extravasate into the tumor interstitium, while poor lymphatic clearance prolongs their retention. Key factors influencing passive targeting are nanoparticle properties such as Size, shape, surface charge, and hydrophilicity affect tumor accumulation. Smaller AuNRs with high aspect ratios (AR) and biocompatible coatings (e.g., PEG, proteins) exhibit prolonged circulation and better tumor uptake. In recent years, advances have been made in passive targeting using gold nanorods. A novel nanocarrier, DNA-origami nanostructures (e.g., triangular DNA carriers), loaded with AuNRs, facilitated the accumulation of drugs in the tumor tissue [114]. Moreover, hydrophilic polymers (e.g., PEGylation), proteins, and stealth coatings are usually conjugated to the surface of AuNRs, improving the EPR effect by prolonging systemic circulation [115]. However, this strategy has limitations due to the EPR effect is highly variable, limiting therapeutic predictability. Also, passive targeting lacks specificity, leading to off-target effects [116].
Active targeting enhances tumor delivery by functionalizing AuNRs with ligands such as antibodies, peptides, aptamers, or vitamins that bind to overexpressed receptors on cancer cells or within the tumor microenvironment (TME), promoting receptor-mediated endocytosis [117–119].
To overcome the disadvantages of natural antibodies, such as poor stability, comparably the complicated preparation process, and low affinity toward nonimmunogenic targets, molecular imprinting technology was exploited to construct sialic acid (SA)-imprinted AuNRs, which exhibited high affinity to cancer cells overexpressing SA [120–122]. Additionally, increasingly more studies have focused on the potential molecular targets in TME. Serum albumin, capable of binding to gp60 receptors expressed on tumor vascular endothelial cells, was added to AuNRs-based nanomedicines to achieve long blood circulation time and more intracellular accumulation of chemotherapeutic agents [123]. The advantages of active targeting are higher cellular uptake compared to passive systems and also enable deeper tumor penetration (e.g., enzyme-triggered shedding of stealth coatings) [124]. However, this strategy has limitations due to ligand-receptor binding does not always increase total tumor uptake but redistributes nanoparticles within the TME [125]. Also, hypoxia and enzyme expression vary between tumors, requiring multi-targeting approaches [126].
Efforts to improve the clinical translation of gold nanorod-based photothermal therapy (PTT) focus on surface modifications for enhanced biocompatibility and stability [127], improved tumor targeting to increase therapeutic precision [128], combination therapies for synergistic effects [10], and minimizing off-target toxicity to enhance safety and efficacy [8]. Nevertheless, several limitations hinder the clinical translation of AuNRs. Cytotoxicity cetyltrimethylammonium bromide (CTAB) used in AuNR synthesis [129], inducing ROS created during laser irradiation that leads to oxidative stress and DNA damage [130, 131], aggregation bare AuNRs in physiological fluids and rapid coating by serum proteins [132], triggering clearance by the mononuclear phagocyte system (MPS) [133], slow biodegradation AuNR accumulation in organs (e.g., liver, spleen) [3], raising long-term safety concerns.
Researchers have developed strategies to improve the clearance and excretion of AuNRs. These approaches focus on modifying the physicochemical properties of AuNRs and leveraging biological mechanisms to enhance their elimination. 1) Surface modification for improved biocompatibility and clearance, such as coating AuNRs with PEG [134], encapsulating AuNRs in degradable shells (e.g., mesoporous silica) [135], and enzyme-cleavable coatings [136] that can facilitate faster excretion and degradation in specific tissues. 2) Size and shape optimization, such as reducing the aspect ratio, which is more easily filtered by the kidneys. 3) Stimuli-responsive disassemblies such as pH- or redox-sensitive linkers that cause to breakdown of AuNRs designed in the acidic environment of lysosomes or under redox conditions, resulting release of smaller fragments for excretion [137, 138]. Also, laser irradiation can melt AuNRs into smaller spherical particles, aiding clearance [139].
The synthesis of gold nanorods (AuNRs) faces several hurdles in large-scale production and reproducibility, including complex synthesis conditions, inconsistent yields, and cost and environmental concerns. Small changes in reagents (e.g., cetyltrimethylammonium bromide (CTAB) or silver ions) can drastically alter gold nanorods’ morphology (aspect ratio, yield) [140, 141]. Purification difficulties are also other challenges in the synthesis of AuNRs. Removing excess surfactant (CTAB) and byproducts (spherical nanoparticles) at large scales is challenging and affects reproducibility [142]. Minor variations in reaction conditions can cause problems in reproducing gold nanorods. Temperature fluctuations, mixing rates, and impurities can lead to inconsistent aspect ratios and optical properties. In addition, CTAB, a critical stabilizing agent, often varies between suppliers, affecting nanorod uniformity [143].
AuNRs’ stability depends on surface chemistry, environmental conditions, and external stimuli [141, 144]. AuNRs are typically stabilized by surfactants like CTAB or coated with polymers (e.g., PEG, PSS) or silica shells. The CTAB provides short-term stability but can desorb over time, leading to aggregation. In addition, the tips of AuNRs are more reactive due to high curvature and may undergo oxidative dissolution, especially in the presence of reactive oxygen species (ROS) or halides (e.g., Cl⁻) [144]. Etching agents like strong oxidants (H₂O₂) can degrade AuNRs, shortening their aspect ratio and shifting their SPR peak [145].
They degrade over time via oxidation, aggregation, or reshaping. AuNRs may shorten or become spherical due to surface atom diffusion or chemical etching. Also, the loss of stabilizing ligands leads to particle clustering, broadening the SPR peak. Oxidation (e.g., by dissolved O₂ or ROS) converts Au0 to Au3+ ions, reducing particle size [146].
By addressing these challenges, AuNR-based PTT holds great promise for precise, effective cancer therapy with minimized side effects.
CONCLUSION
The AuNRs have emerged as a transformative tool in the field of cancer therapy, particularly in PTT and synergistic chemo-photothermal approaches. Their unique optical properties, including strong absorption in the NIR region, enable efficient conversion of light into heat, allowing for precise and localized destruction of cancer cells while sparing healthy tissues. Functionalizing AuNRs with targeting molecules (ligands) and drugs (therapeutic agents) to gold nanorods (AuNRs) can improve drug delivery and controlled release. This helps overcome key challenges in cancer treatment, such as drug resistance and off-target effects. Recent advancements in surface modification and functionalization have significantly improved the biocompatibility, stability, and therapeutic efficacy of AuNRs. Innovations such as the removal of cytotoxic surfactants like CTAB, the incorporation of stimuli-responsive polymers, and the development of multifunctional nanocomposites have expanded their applications in cancer therapy. These advancements have enabled the integration of AuNRs into sophisticated drug delivery systems, where NIR-triggered hyperthermia and drug release work synergistically to enhance therapeutic outcomes. The combination of PTT with chemotherapy has shown remarkable promise in overcoming the limitations of conventional treatments, particularly in resistant cancers. Systems such as USGRV–17–AAG, (DOX-OA+VERA+AuNRs)@NLC, and CPT + AuNRs@NSLCs demonstrate the potential of AuNRs to deliver both heat and chemotherapeutic agents in a controlled manner, significantly improving tumor ablation and reducing recurrence. Furthermore, the development of sequential drug delivery systems and pH/NIR dual-responsive platforms highlights the versatility of AuNRs in addressing the complex tumor microenvironment.
In conclusion, gold nanorods represent a powerful and versatile platform for advancing cancer therapy. Their unique properties, combined with ongoing innovations in nanotechnology, hold immense potential to revolutionize cancer treatment by offering minimally invasive, highly targeted, and effective therapeutic strategies. As research continues to refine their design and application, AuNRs are poised to play a pivotal role in the future of oncology, bringing hope for improved outcomes and quality of life for cancer patients worldwide.
STATEMENTS AND DECLARATIONS
Authors' contributions
Maryam Deinavizadeh performed this study, conceptualization, and article writing; Ali Reza Kiasat performed this study, writing—review and editing, and supervision; Roya Mirzajani performed the methodology and validation; Mohammad Shafiei contributed to the literature review; Mohammad Sabaeian conducted data analysis, and Pooyan Makvandi revised the manuscript.
Competing Interests
The authors declared no potential conflict of interest.
Ethics approval
Not applicable.
Data availability
Not applicable.
Funding
No funding was received to support this work.
ACKNOWLEDGMENTS
This work has been supported by the Center for International Scientific Studies & Collaboration (CISSC), Ministry of Science Research and Technology of Iran.
AUTHORS’ INFORMATION
Maryam Deinavizadeh—Department of Chemistry, Faculty of Science, Shahid Chamran University of Ahvaz, Ahvaz, Iran;
Ali Reza Kiasat—Department of Chemistry, Faculty of Science, Shahid Chamran University of Ahvaz, Ahvaz, Iran;
Roya Mirzajani—Department of Chemistry, Faculty of Science, Shahid Chamran University of Ahvaz, Ahvaz, Iran.
Mohammad Shafiei—Department of Biology, Faculty of Science, Shahid Chamran University of Ahvaz, Ahvaz, Iran.
Mohammad Sabaeian—Department of Physics, Faculty of Science, Shahid Chamran University of Ahvaz, Ahvaz, Iran; Center for Research on Laser and Plasma, Shahid Chamran University of Ahvaz, Ahvaz, Iran.
Pooyan Makvandi—Centre of Research Impact and Outcome, Chitkara University, Rajpura 140401, Punjab, India;