Visual Abstract
Abstract
Freshwater comprises only 0.5% of Earth's water and is increasingly threatened by population growth and industrial activities, making water scarcity a critical issue. Membrane separation technology is gaining attention as an effective method for water purification, utilizing specially designed membranes that allow water to pass while blocking pollutants, thereby saving energy and reducing chemical use. Recent advancements in composite membranes, which integrate inorganic porous materials with polymers, have improved their permeability and selectivity for diverse water treatment applications. Metal-organic frameworks (MOFs) are notable for their tunable pore structures and high surface areas, enhancing wastewater treatment effectiveness. This review discusses water-stable MOF-based membranes' design, synthesis, and properties, emphasizing their potential for removing heavy metals, dyes, oil, and organic contaminants. However, challenges remain, particularly regarding the compatibility of MOFs with polymer matrices, underscoring the need for further research to optimize their stability and performance in addressing global water scarcity.
Introduction
Whereas water covers 72% of the land surface, just a tiny portion of these water sources—roughly 0.5% are regarded as freshwater sources [1]. Due to the population’s perpetual and exponential increase, the changing environment from ancient times to the present, and the many careless operations of companies and the urban sector, water resources are becoming more limited. Therefore, it is imperative to find a solution to the water scarcity issue since it is a valuable and vital resource [2].
Over the years, several strategies and approaches to handle and conquer this issue have been proposed, but water pollution control employing separation technology can be one of the most effective approaches to water scarcity [3]. Membrane separation, which relies on membranes with pores capable of selecting molecules, is of great interest in the water treatment industry because of its low energy consumption compared to other separation methods. This method is preferable because the membrane’s pores can selectively filter out pollution molecules while allowing water molecules to pass through it, without changing the phase or adding chemicals [4,5]. As a result, membrane separation, which has been considered as a viable solution for addressing energy and environmental concerns, has advanced dramatically in recent decades. Membranes make it easier to separate, purify, and concentrate different feed liquid components via selective separation, but it is important to strike a balance between permeability and selectivity [6].
By incorporating inorganic porous materials into the membrane matrix, the researchers were able to alter the size and configuration of the membrane pores, hence increasing the permeability and selectivity of the fabricated membranes. To combine the benefits of the favorable permeability of the polymer phase with the high selectivity of the inorganic phase, a new generation of membranes termed as composite membranes was created [7]. Despite this benefit of composite membranes, there has been a considerable rise in the search for the production and design of inorganic additives owing to poor compatibility between inorganic additives and polymer phase and interphase repulsion and loss of membrane porosity [8]. Furthermore, membrane materials play an important role in membrane separation and come in a variety of forms. It’s essential to choose a membrane material with appropriate selectivity, permeability, and antifouling characteristics. Consequently, it is necessary to select an appropriate substance that can serve not only as an amazing additive but also as a raw material for creating good membranes [9].
MOFs, which are made up of metal ions and bridging organic ligands, have been proven to perform better in membrane separation. There are a few distinct MOF structures that may be used for various purposes such as Zeolitic imidazolate framework–71 (ZIF–71), Zeolitic imidazolate framework–68 (ZIF–68), Zeolitic imidazolate framework–67 (ZIF–67), Zeolitic imidazolate framework–11 (ZIF–11), Zeolitic imidazolate framework–8 (ZIF–8), aminated metal‐organic framework–53 (MIL–53 (Al)), MIL–125, MIL–101 (Cr), and Universitetet i Oslo (UiO–66) [10,11]. Various metal clusters’ typical MOF structures were shown in Figure 1. In the past fifteen years, metal-organic frameworks, or MOFs, have been the subject of extensive research and have emerged as one of the most intriguing classes of materials for scientists and engineers. This is based on that MOFs possess advantageous properties such as regular and tunable pore structures and abundant adsorption sites [12].
Nowadays, although membrane processes are widely used in the industrial sector for wastewater treatment and water regeneration, MOF-based membranes were mostly sought for gas storage and separation and have not gotten as much attention in water treatment as they might have [13,14]. Water regeneration and the application of MOF membranes in wastewater treatment are both progressively expanding. MOF may be used as fillers in mixed matrix membranes (MMM) and thin-film nanocomposite (TFN) membranes for wastewater treatment and water regeneration. To create bare MOF membranes, MOF may also be employed as matrices [15–17].
There are many different forms of MOF; however, water stability is a need for MOF to be used in wastewater treatment and water regeneration [18]. Only MOF materials that are stable in water—that is, do not structurally break down when exposed to water—are discussed in this chapter. This chapter scrutinized the design and synthesis methods of MOF-based membranes, the characterization of MOFs, as well as the use and properties of MOF-based membranes for a variety of applications, including the rejection of dyes, salts, heavy metals, organic pollutants, and PFAS.
The development and implementation of metal-organic framework (MOF)-based membranes for water decontamination depend on several factors, including material stability, performance improvements, and the ability to scale for industrial use. Recent advancements show a promising outlook for MOF membranes, with ongoing research focused on overcoming current challenges. To enhance understanding of different types of MOF-based membranes, a brief summary of their development timeline for separation applications is presented in Figure 2. [23].
Previous reviews have explored the removal of contaminants of emerging concern (CECs) from municipal wastewater utilizing metal-organic framework (MOF)-based membranes. These studies primarily emphasized engineered and synthesized strategies aimed at reducing fouling and enhancing water permeability [24, 25]. In contrast, this review provides an overview of various synthesis methods, current rational design methodologies for water-stable MOF membranes, followed by a discussion of MOF chemical characterization. Furthermore, the most recent uses of MOF-based membranes are discussed in detail and the removal of each aquatic pollutant (especially in recent years) is explored separately. Due to MOF membranes’ superior permeability, selectivity, and antifouling capabilities, it has been shown that they are attractive options for wastewater treatment and regeneration, and further research into their potential application in wastewater treatment and regeneration is warranted. By emphasizing the practical implications of MOF research and its potential for addressing real-world water treatment issues, this work offers a fresh and valuable perspective that can contribute significantly to the existing body of literature.
MOF-based membranes
Before, we defined MOF-based composite membranes as those in which micro or nano particles of MOFs were incorporated into a polymer matrix. The addition of MOF particles to polymers not only helps polymeric membranes overcome the inherent trade-off that exists between their permeability and selectivity, but it also improves the membranes’ mechanical strength and thermal stability [26]. In the following, we will discuss the synthesis of MOF particles as well as the synthesis strategies of the two most important types of MOF composite membranes, viz., TFN and MMM.
Synthesis procedures of MOFs
Synthetic methods for MOF have been developing rapidly over the last two decades, with developments in Solvothermal, Ultrasound (US) and Microwave (MW), Mechanochemistry, Continuous flow manufacturing, and Electrochemical techniques. Additionally, some researchers are interested in the use of MOF as fillers in polymeric membranes for the treatment of water. Following is an explanation of each process for producing MOFs [11,27,28].
Solvothermal procedure
Extensive research has been done on the solvothermal approach, which is widely recognized as one of the most efficient and widespread techniques for preparing MOF across all size ranges. Because of the nature of the solvothermal synthesis process, reactions must take place in a sealed container at an autogenous pressure that is higher than the threshold at which the solvent would boil. Important reaction conditions including volume, concentration, temperature, and time must be managed. The solvothermal approach relies on careful deliberation about the choice of the chemical modulator as its central component [29,30]. This is of the utmost importance. When different amounts of modulators are added, the sizes of the crystallites change. This happens because the competition for metal-binding sites changes the number of nucleation sites [31,32].
US and MW procedures
As an alternative to conventional heating methods, US and MW approaches have been intensively studied because of their potential to allow rapid crystallization of MOF. Furthermore, MW makes the nucleation process more quick and uniform, leading to more uniform particle size distributions, as a consequence of the creation of local super-hot spots and rapid heat transmission [10,11].
Mechanochemistry procedure
Mechanochemistry is a top-down technique used to reduce the size of MOFs by grinding them after they have been synthesized. To generate a large quantity of fine granular MOF in 10–60 minutes, this solvent-free green synthesis approach is regarded as advantageous because of its high yield and rapid reaction time. Microcrystalline powders of MOF may be produced using this solid-state thermomechanical technique, which seems to apply to all MOF types [33,34].
Continuous flow manufacturing procedure
By dramatically increasing the surface area to volume ratio, the continuous flow technique improves heat transmission and mass transfer in the reaction system, allowing for quick and efficient synthesis and the eventual possibility of mass manufacturing of MOF materials [35,36].
Electrochemical procedure
Instead of using metal salts, anodization is used to create metal ions in electrochemical synthesis. So, the metal source doesn’t have to cause the anion interference that would have happened otherwise. Metal ions created locally at the support surface might impede crystal growth while also transporting byproducts that aren’t useful. At standard temperatures and pressures, electrochemical synthesis is possible, and it offers controllable conditions like voltage and pulse signal. The application of electrochemical techniques in the industry is thus widespread [37,38].
Fabrication procedure of TFN membranes
The vast majority of the time, TFN membranes are made out of polymers and are supported by polymers. First created by Jeong et al. In actuality, a polyamide (PA) film that includes nanoparticles and is supported by a plastic sheet, such as polysulfone (PSU) or polyvinylidene fluoride (PVDF), is a popular TFN membrane for water filtration [39]. Initially, Sorribas et al. inserted MOF as an additive to TFN membranes. The reticulated porous architectures, equal pore widths, and nanoscale particle sizes made it more receptive to polymer chains than other forms of nanoparticles. Interfacial polymerization (IP) is the process that is often used to produce TFN membranes [21]. IP refers to the polycondensation reaction that takes place at the interface (or the organic phase side of the interface) of two mutually incompatible solutions in which two monomers are dissolved [40].
Fabrication procedure of MMM
MMM is a composite membrane consisting of a polymer matrix (the continuous phase) and micro-or nano-particles (the discrete or dispersed phase; also known as additive) of an inorganic or inorganic-organic hybrid material [41]. In addition to being very simple and affordable to scale up, the MOF-based MMM is also more stable [42]. The blending method is one of the most popular and crucial approaches to fabricating MOF-based MMM, and it can be further subdivided into two types: blending on a substrate and blending without a substrate. In general, the substrate-based blending process consists of three steps: Spin-coating, dip-coating, or flat membrane casting the solution containing the MOF and polymer into the porous support, and then removing the casting solvent during solidification or desiccation, which are the three methods often used to create MOF ink [11,43,44].
Substrate-free blending allows for more design freedom and simplified delamination of nonporous supports from the substrate than their supported counterpart. For optimal mechanical strength and permeability, the resultant membranes’ thickness is typically kept within a range of 18–30 mm [45,46].
Water treatment via MOF-based membrane
Powerful approaches are needed for water treatment in the twenty-first century because mainly pollutants are not simple components, or the source of pollutants could be unknown, and the multi-contaminants are a huge obstacle in front of water treatment [47,48]. Hence, versatile methods are desirable, and materials with multifunctional applications are getting more eyes. Having said that, in previous sections, MOFs are multifunctional materials because they are deadly tunable, so they could be used in a sieving mechanism. On the other hand, most of them possess many functional groups, which are active sites, so they could be used as adsorbents too. Besides, polymeric composites can surpass the MOFs regarding efficiency and total cost. Therefore, MOF-based membrane is a promising approach for water treatment, but they are not flawless. Inorganic nanomaterials have poor compatibility with an organic texture like polymers, meaning MOF-based membranes suffer from a lack of long-term stability [27,28,49]. Recently, dual modification or modified MOFs have been reported as alternative ways to unravel this drawback. Core-shell MOFs with an organic shell cannot only augment the surface ratio of MOFs but also solve the low compatibility simultaneously. Different cores require different shells, and this solution has its limitations [50].
There are several ways to sort MOF-based membranes for water treatment, like sorting based on the fabrication methods or type of MOF and polymeric matrices. However, in this review, the sorting is based on the pollutants. The goal is to emphasize that choosing the suitable membrane for the correct application can save energy and time.
Removing anionic components
Active components can cause detrimental damage to humans and the environment. Components like fluoride (F) ions can be dissolved into the water quickly, which means they can be even more toxic. The major problem for removing fluoride solution is the stability of materials in this condition because fluoride could damage their structure, and it is a corrosive agent [51,52]. MOF-based membranes are among the materials that can maintain their structure in the fluoride solution. The MOFs’ tunable porosity, metallic nodes, and coordination numbers are obvious fortes regarding Fluoride removal. In addition, MOFs with hydroxyl and amine groups revealed more capacity for removing anionic components, and those with Zr or Cr cores indicated promising efficiency [53,54]. Hence, UiO–66 (Zr) is an appropriate candidate for this type of pollutant. It was reported that in-situ growth of UiO–66 and its derivatives on the polyethylene terephthalate (PET) surface has an excellent removal capacity for fluoride removal. As can be seen from Figure 3, the Amino or N(CH3)3 derivatives of UiO–66 can form selective and highly conductive channels. The Zr sites on the UiO–66 showed a significant tendency toward F- ions compared to others, indicating selective fluoride removal with riveting efficiency. Moreover, the tests revealed that the removal efficiency of this MOF-based membrane was in this order: F->Cl->Br->I->NO3->SO42-. The mentioned paper and other similar studies revealed that MOF-based membrane is a low-energy consumption method with ruthless efficiency, which can be used in an anionic separation application [55]. Creating ion channels in MOF-based membranes could be a fantastic vehicle for further research.
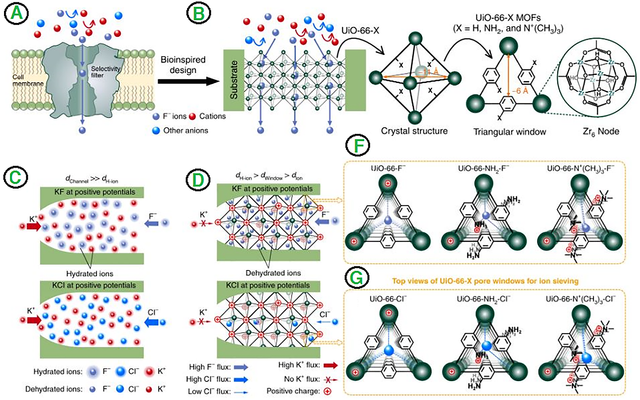
Desalination
Earth is known as a blue planet, and most of its surface is covered by water, but many countries and people suffer from a lack of drinkable water. Desalination has a long history, and boiled water is probably the oldest way, even though it is not economical. The human population is growing at a staggering pace, and at this rate, using desalination is inevitable for many countries around the world. Using a membrane for desalination is a promising method because it requires low energy and is cost-effective [16,17]. MOF-based membranes have been used for desalination for almost two decades because they can offer many merits. The porous nature with significant tunability, high thermal stability, multifunctional groups, and high surface ratio make most MOFs materials with high removal capacity for desalination. Different MOFs like MILs, ZIFs, and UiO–66 have been used for salt removal [56,57]. These nanomaterials were added to polymeric matrices or between the PA layers solely or conjointly with other particles, resulting in composites used for desalination. Even though microfiltration, ultrafiltration, and loose nanofiltration membranes are not appropriate for desalination, tight nanofiltration, reverse osmosis, and forward osmosis membranes have been used regularly for the purification of water from different salts. In the membrane field, continuous pressure is an absolute need for operation. This pressure is even higher for membranes for desalination purposes because they mostly have smaller pore sizes. This high pressure can wash out MOFs from the composites’ structure and reduce the membranes’ consumable life. Hence, particular linkers, post-treatment and dual modification methods are required to fabricate composites with appropriate longevity [58–61]. Different ZIF were incorporated into polymeric matrices to form RO membranes, and their desalination performance was investigated. All used ZIF had the same rho-topology, so the result indicated that different linkers could make a drastic difference in the outcomes [62]. Conversely, researchers used an in-situ approach for growing MOF particles on the transition metal carbides (MXene) nanosheet layers, introduced the resulting heterostructures into the PA layers, and fabricated a tight nanofiltration membrane for desalination. The synthesized heterostructure in this study showed excellent MOF stability in the polymeric matrices. The removal capacity significantly improved, and its efficiency reached as high as 97.5% for Na2SO4. As shown in Figure 4, the fabrication steps of thin-film composites can be seen where PVDF is a porous support [6].
MOF-based membranes for desalination presents several challenges that need to be addressed for effective implementation. These challenges primarily revolve around the balance of permeability and selectivity, structural stability, and the integration of MOFs with existing membrane technologies. Achieving high permeability while maintaining selectivity is crucial. For instance, ZIF–8 matrix nanocomposite membranes have shown superior permeability (>43.6 LMH bar−1) but require careful design to ensure high salt rejection (∼95.1%). The challenge lies in increasing MOF loading without compromising the interfacial compatibility with polymeric matrices, which is essential for optimal performance [63].
MOF membranes must exhibit stability under harsh conditions. Ceramic-based MOF membranes have demonstrated high water flux and near-complete salt rejection, indicating their potential for challenging desalination applications. The integration of MOFs with ceramic membranes enhances their physicochemical properties, contributing to their durability [64].
Heavy metal removal
Since the industrial revolution in Europe, the number and type of industries have risen drastically. The outlet wastewater of such industries contains a variety of pollutants that are harmful to both humans and the environment. The outlet of wastewater in the industries like batteries, mining, automobile manufacturing, tanneries, and others possesses a certain amount of heavy metals, and purifying the wastewater of these industries is a critical must. It is worth mentioning that most industries are convenient to primary water sources like rivers, lakes, seas, and oceans; therefore, water pollution is decisive [65].
The possible question is what are heavy metals? This is a tricky question because different countries and organizations have different definitions of this matter. However, almost all agree that metals with 60–200 nm atomic radius are considered heavy metals. Most heavy metals are stable and non-degradable in the environment, so their existence has irreversible consequences, so removing them is a priority. Metallic ions like mercury, copper, lead, selenium, chromium, nickel, cobalt, and arsenic are the most common heavy metals, and removing them from water is getting more attention. Even low concentrations of arsenic can lead to skin cancer or lung damage. Selenium is known for severe damage to kidneys and even death. Mercury is detrimental to the brain and lungs. At the same time, chromium, nickel, and cobalt are deadly agents for the liver and can cause severe dehydration and result in excessive vomiting. Treating these illnesses sometimes is impossible and requires a vast amount of time and budget; besides, there is a chance that they can affect other generations through genes [61,66].
A promising approach for removing heavy metals would be a method that shows high efficiency and prepares a condition to recover those heavy metals. Recovering heavy metals or their oxide forms is economical and among the hottest topics. One of the supreme approaches for heavy metal remediation is using MOF-based membranes because MOFs are highly porous and have an excellent surface area resulting in remarkable removal capacity. Facile functionalization is their other merit. Moreover, changing the linkers of MOFs means changing the functional groups and fabricating a specific is feasible. On the other hand, integrating MOFs into polymeric or ceramic matrices can enhance their flexibility and processability [3,67].
Hexavalent chromium (Cr6+) is one of the most toxic heavy metals and its toxicity is more than 400 times that of Cr3+, so purifying it from water has been investigated by many researchers worldwide. A wide range of MOF-based membranes was used to remove Cr6+ and most of them revealed high removal capacity (6 mg/g or more) [68,69]. Based on the EPA or the American protection agency, 0.002 mg/L of Hg2+ or more is toxic and needs special treatment, adsorption of this metal via active sites of MOF-based membranes was reported [65]. Cadmium and lead ions have a considerable toxicity and concentration above 0.005 mg/L and 0.05 mg/L of them could bring harm to the humans’ organs and similar to most metals, adsorption via active groups is a key method for removing them from water [66].
Having hydroxyl groups or amine groups is a staple part of fabricating desirable MOFs for removing metallic ions like Pb2+, Cr6+, Zn2+, and other common heavy metals, and a list of recent papers in this regard was brought in Table 1. Generally, coordination interaction between amine and hydroxyl groups is the key reason to enhance the removal capacity and other factors like temperature, metal concentration, fillers concentrations, applied pressure of filtration, and type of MOF-based membrane showed some level of impact on the removal efficiency. Another crucial factor is the pH of the solution because coordination interaction depends on this element. It is worth mentioning that highly acidic or alkali solutions can bring damage to most polymeric and metallic membranes, so this is an obvious drawback of these types of composites in heavy metal removal [39,51]. Rao and colleagues fabricated a thin-film membrane with MOF/graphene oxide fillers to remove heavy metals [70]. Bayrami and colleagues used a Zwitterion functionalized aminated MOF to fabricate thin-film composites and employed them to purify water from heavy metals [9]. Mansoorianfar and his colleagues investigated the usage of MOFs as promising porous materials for removing cadmium from water [71].
Despite the published articles and the mentioned points, there are still challenges in this field. The challenges of heavy metal removal via MOF-based membranes and the synthesis of MMMs utilizing MOFs for size-selective adsorption include (i) stability: Many MOFs can be sensitive to moisture or certain pH conditions, leading to structural degradation, which can compromise their effectiveness in heavy metal removal over time. (ii) Scalability: The synthesis of MOF-based membranes often involves complex procedures that may not easily scale up for industrial applications, raising concerns about the cost-effectiveness and feasibility of large-scale production. (iii) Fouling: MOF membranes can be prone to fouling, where contaminants build up on the membrane surface. This can reduce the membrane’s permeability and separation efficiency, necessitating frequent cleaning or replacement. (iv) Limited Selectivity: While some MOFs can effectively adsorb certain heavy metal ions, their selectivity for specific metals can be inadequate. This can lead to inefficient removal when multiple metal ions are present in wastewater. (v) Recyclability: Although some MOF membranes can be reused, their effectiveness may diminish with repeated cycles. Developing a robust MOF that maintains high performance after multiple uses is a crucial challenge. (vi) Separation Mechanism: Understanding and optimizing the separation mechanisms (adsorption, size exclusion, etc.) at play can be complex, particularly when dealing with a mixture of heavy metals with varying chemical properties. (vii) challenges in synthesizing MMMs utilizing robust defective MOF: it itself include achieving good integration between the MOF and the polymer matrix, ensuring uniform dispersion of MOF nanoparticles within the polymer matrix, striking a balance between the MOF’s adsorptive capacity, cost of raw materials: the production of high-quality MOFs and polymers can be expensive, which can limit the economic viability of MMMs for large-scale applications [72–75].
Membrane title | Heavy metal type | Efficiency | Released year | Reference |
Polyamidoamine Mil 125 (Ti)/PES | Pb2+ | 97.3% | 2022 | [76] |
Uio–66-NH2/PAN-chitosan | Pb2+, Cd2+, Cr6+ | More than 90% | 2019 | [77] |
ZIF–67/MXene-sepiolite membrane | Cu2+ | 80% | 2022 | [78] |
ZIF–8 nanocomposite | Cu2+, Zn2+, Cd2+ | More than 84% | 2022 | [79] |
β-cyclodextrin-ZIF–8/PVDF | Pb2+, Cu2+ | - | 2022 | [80] |
NH2-MIL–53/wood carbon membrane | Pb2+ | - | 2020 | [81] |
UiO–66/polysulfone | Sr2+, Cd2+, Cr6+ | More than 80% | 2022 | [82] |
UiO–66/tannic acid/chitosan/PES | Cr6+ | 99% | 2021 | [83] |
m-phenylenediamine/aminated Uio–66 TFN | Cr3+ | - | 2021 | [84] |
FeBTC@Nylon 6,6 and ZIF–8@Nylon 6,6 membranes | Pb2+, Cd2+, Hg2+ | 2023 | [85] | |
P-SMG@ZIF–8 | Pb2+, Cu2+ | 80.2 %, 65.6 % | 2024 | [86] |
MOF5@GO polysulfone-based mixed matrix membrane | Pb2+, Cu2+, Zn2+and Cd2+ | 95–99 % | 2024 | [87] |
Dye removal
Dyes are inseparable parts of modern life. Although using natural dyes for thousands of years for different purposes, nowadays dyes find themselves in coatings and food to weaving industries. Moreover, textile, machinery, clothing, and many other industries need dyes and pigments and without them, they are simply defective. Organic dyes are the most common ones among dyes, their existence in water or soil has a deleterious effect on the environment, and living creatures because they mainly contain toxic components. Aromatic rings, azo groups, nitro groups, and amine groups are common among organic dyes, which are components known for their carcinogenetic impacts. Therefore, expunging these pollutants from water and outlet wastewater of dyes industries is necessary [88,89]. Adsorbing dyes is one of the key methods to remove dyes from water and got the attention of many researchers during the past few decades. Hence, materials with high surface area and active sites are potent for dye removal, so a MOF-based membrane could be a reasonable method. Moreover, the tunable porosity of MOFs means they can play as a sieve and separate dyes via shape and molecular weight. Functionalized MOFs with hydroxyl and amine groups can adsorb dyes by hydrogen bonds, coordination interactions, or electrostatic interactions [72]. Heidari and his colleague used MIL–53/PES mixed matrix membrane for removing four aqueous dyes with remarkable efficiency [90]. Vatanpoor and his colleagues used a contra-diffusion synthesis approach to form a uniform layer of ZIF–8 on the PVDF surface and used the resulting composites for the remediation of dye from wastewater with enhanced permeation [84]. Mahdavi and his colleagues used a dual modification method to fabricate a desirable composite for the purification of water from dyes. They used NH2-MIL–101 (Al) and H2O2-treated exfoliated graphitic carbon nitride (C3N4) conjointly for modification of the PVDF membrane and reported the synergistic effect of them on each other. As shown in Figure 4, a vapor-induced phase separation (VIPS) method was used for the fabrication of the final modified membrane where functionalized C3N4 plays as an anchor for aminated MOF and led to the long-term stability of the prepared membrane [7].
Two-dimensional bimetallic MOFs, such as FeNi-MOF, are engineered into heterostructure membranes, promoting both separation and catalytic degradation of dyes. In one research, a two-dimensional bimetallic MOF that serves as a heterogeneous Fenton-like catalyst, which can be engineered into lamellar membranes. This MOF is combined with ultrathin g-C3N4 spacers to prepare a heterostructure membrane. The construction of the heterostructure involves alternating the arrangement of FeNi-MOF and g-C3N4 nanosheets, utilizing coordination bonds between bimetallic nodes and pyridinic nitrogen. The membrane effectively traps contaminant molecules through specific or non-specific interactions within the nanochannels, while radicals generated within the membrane degrade the contaminants, achieving a dye removal rate of approximately 99.0% [91].
In other study, a robust MOFs material named Al-UiO–66 was synthesized by doping Al into UiO–66 material through one-step in-situ synthesis method, which increased the pore size distribution of MOF material. Al-UiO–66 nanoparticles were blended within PVDF casting solution. The researchers tested the membrane’s ability to remove six different dyes with varying charges and sizes, including cationic dyes like Malachite Green and Rhodamine B, as well as anionic dyes such as Methyl Orange and Reactive Black 5. The results were striking: the Al-UiO–66-MMM achieved over 99% removal rates for several smaller dyes (Methyl Orange, Methyl Yellow, and Malachite Green), while it showed lower adsorption for larger dyes, indicating a size exclusion effect. When separating mixtures of dyes, the membrane consistently removed around 99% of the target dyes, showcasing excellent selectivity. In terms of durability, the Al-UiO–66-MMM could be reused effectively, maintaining over a 90% removal rate after 10 cycles of filtration with high selectivity [92]. Some of MOF-based membranes for the dyes removal are presented in Table 2.
Membrane title | Dye type | Removal efficiency | Reference |
ZIF–8/PEI hybrid NF | CR | 99.2 % | 93 |
Cu-MOF- CMFP | RhB MB MG | 90.7 % 91.6 % 87.8 % | 94 |
PCN–224/TA/PVDF | MB RhB | 98.5 % 99.4 % | 95 |
Zr-MOFs-PUF membrane | RhB, MB, CR | 98.80 %, 97.57 87.39 % | 96 |
Ag@AgCl@MIL–100(Fe)/CCF membrane | soluble dye adsorption ability | 99.23 % | 97 |
Al-UiO–66-PVDF | Methyl Orange and Malachite Gree | over 99% | 98 |
Sm-MOF/GO membrane | RhB | over 91% | 99 |
MIL–53(Fe) ultrafiltration membranes | Methylene Blue | 86% | 100 |
Oil removal
The existence of oil in the water could lead to an imbalance in nature because it could damage marine creatures and lead to severe agglomeration in the plant’s vessels, besides there are numerous reports about the detrimental effects of exorbitant oil on the soil [101]. Burned fuel oil possesses numerous toxic components like radical carbons, nitro groups, and sulfur groups. Although air pollution is the major issue regarding burned fuel oils, disposing of the burned oil could lead the water pollution and with the rising in the vehicles number, the oil removal from water is getting more attention there are some reports about the possibility of recovering burned oil and reusing it [102]. Moreover, with the rising human population and remarkable shifting in food style the usage of edible oils saw a drastic raise, which means oil pollution in municipal wastewater is a serious concern in the recent century. Removing sunflower, canola, olive, palm oil, soy oil, and margarine was part of numerous research in oil/water separation during recent years [103,104]. MOF-based membranes were used for oil removal occasionally because they can remove oils with two mechanisms, which are adsorption/desorption and sieving. The pore size of most MOFs is smaller than conventional oil size so their channels can be appropriate routes for the passage of water molecules without letting oil molecules pass through [105].
In addition, microfiltration and ultrafiltration membranes were widely used for the remediation of oils because they can remove oils with high efficiency without sacrificing permeation rate while nanofiltration and osmosis membranes have a lower permeation rate so they are not normally used for this purpose [105]. In the field of oil-water separation, traditional membrane materials often struggle with low permeability and quick fouling, which limits their effectiveness. To address this, researchers are increasingly looking at super-wettable materials for treating oily wastewater. Metal-organic frameworks (MOFs) have emerged as promising options in separation technologies, but their application in oil-water separation has been hindered by issues related to their stability in water and the difficulty of creating durable thin layers on support materials. Wang and his colleagues fabricated a beetle-like membrane consisting of hydrophobic/oleophilic ZIF–8 bumps and polyacrylonitrile (PAN) with a more than 99.9% rejection rate [106]. Shi and his colleagues introduced ZIF–8 nanomaterials into poly lactic acid (PLA) to fabricate nanocomposite electrospun membrane for excellent oil/water separation and the prepared membrane not only revealed high mechanical stability but also showed high oil/water separation because of its fast oil-wetting ability [107]. Ding and his colleagues used a layer-by-layer (LBL) method for synthesizing continuous nanothickness MOF coatings on the polymeric substrate and used for oil removal from water. In the first step, the polymeric substrate was treated via dense Ɣ-irradiation to form as much as possible reactive groups on the surface to boost the formation of continuous nanothickness MOF [108]. This study introduces a new MOF called KFUPM–100, which is designed to be water-stable and utilizes pivalic acid and adenine as ligands, with copper as the metal component. The KFUPM–100 MOF was successfully grown on a copper mesh using an electro-assisted method, resulting in a membrane that exhibits excellent superhydrophilicity and underwater superoleophobicity properties that make it ideal for separating oil from water. The KFUPM–100@Cu-mesh membrane showed impressive stability, as confirmed by various tests, and achieved over 99% oil rejection with a high permeate flux of 47,808 to 49,300 L/m² h, surpassing the performance of previously reported membranes. Notably, this membrane maintained its effectiveness even after being submerged in water for 10 months and enduring 14 cycles in both acidic and basic conditions. Overall, this research marks a significant step forward in developing hydrolytically stable MOF-based membranes by optimizing the host surface and incorporating a sterically hindered co-ligand, paving the way for new applications in water treatment [109]. In other study, Tannic Acid Modified ZIF–8 (TA@ZIF–8) Composite Membrane was prepared to enhances its performance by altering the structural and chemical properties of the ZIF–8. The membrane is produced using a vacuum deposition strategy, which is a method that allows uniform coating of materials onto a substrate. This technique is efficient and straightforward, facilitating the creation of the composite membrane with desired properties. TA adds numerous hydroxyl (–OH) groups to the surface of ZIF–8, which play a crucial role in enhancing the membrane’s interaction with oil droplets. The formation of hydrogen bonds between the emulsion droplets (tiny oil droplets suspended in water) and the hydroxyl groups on the membrane surface disrupts the interface of the oil droplets. This interaction promotes the collision and merging of smaller droplets into larger ones, making it easier to separate the oil from water. The composite membrane exhibits a separation efficiency with an impressive permeance of 3306 L·m⁻²·h⁻¹·bar⁻¹, indicating its ability to filter large volumes of liquid at high rates. It achieves an outstanding oil rejection rate of over 99.8%, demonstrating its effectiveness in removing oil from water under various conditions [110].
The prepared composite indicated high oil-water separation without sacrificing the water permeation rate. Some recent papers about oil-water separation via MOF-based membranes were listed in Table 3.
Membrane title | Oil removal efficiency | Released year | Reference |
MXene@UiO–66-(COOH)2/nylon supported | More than 95% | 2020 | [111] |
NH2-UiO–66@GO/poly lactic acid | 99.9% | 2020 | [112] |
UiO–66-NH2@PAN composite | More than 95% | 2020 | [113] |
2-hydroxyphosphono acetic acid UiO–66@polyester | 99.8% | 2022 | [114] |
Biomimic polytetrafluoroethylene@ZIF | 99.99% | 2023 | [115] |
Janus ZIF–8@poly(acrylonitrile-co-methyl acrylate) | More than 97.5% | 2023 | [116] |
Carbon fiber nonwoven@PDMS-ZIF–8 | More than 95% | 2021 | [117] |
Photo-Fenton PVDF@NH2-MIL–88B (Fe) membrane | 99.4% | 2020 | [118] |
MIL–53-OH@polyacrylonitrile/polyethyleneimine | More than 99% | 2021 | [119] |
PFAS/PFOS removal
Water pollution has many faces, in the eyes of most ordinary people, the transplant water is drinkable and water with mire is a polluted one. In addition, dyes, oils, heavy metals, and salts are the only pollutants but that is not true. Perfluorooctane sulfonic acid (PFOS) and polyfluoroalkyl substances (PFAS) are among the contaminant that reel in more attention these days because they are part of many components, products, and industries so their leakage in the water is inevitable. From shampoos and tooth floss to cleaning products PFAS/PFOS, are everywhere and they are harmful to humankind because they can reduce fertility, increase the risk of asthma and thyroid disease, augment prostate cancer, and damage the liver, therefore PFAS removal is necessary [120]. The removal of per- and polyfluoroalkyl substances (PFAS) using metal-organic framework (MOF)-based membranes has emerged as a promising solution due to their high efficiency and selectivity. Although PFAS is a general term and consider a class of pollutants rather than a specific component, negative surface charges and fluoride groups are common in all of them. Using different types of catalysts and approaches based on the degradation of organic components are major methods for PFAS removal until this day, but several others methods have been reported as well [120,121]. MOF-based membranes are powerful adsorbents with great removal capacity and they can adsorb PFAS via coordination interaction, hydrogen binding, and electrostatic interaction, besides MOF-based membranes, have a great processability. Zhang and his colleagues used aluminum fumarate metal-organic framework to cure landfill leachate, which is one of the most complicated wastewaters with numerous pollutants like PFAS. They coated poly(vinyl alcohol) (PVA) on the polytetrafluoroethylene (PTFE) and incorporated the Al-based MOF into the hydrophilic layers of PVA to overcome the low permeation rate of membrane and the prepared composite showed more than 99% efficiency for PFAS removal [122]. Li and his colleagues investigated the mechanism of PFAS removal via MOF-based materials. They investigated three MOFs with different shapes and sized and found that the removal mechanism is governed by electrostatic interaction and acid-base interaction for anionic and non-ionic PFAS respectively. Besides this study revealed that MOFs could remove long-chain PFAS more effectively compared to short-chain PFAS [123].
Recent studies highlight the advantages of MOF membranes, including their ability to effectively target both short- and long-chain PFAS, which are notoriously difficult to remove from water. PFAS are highly stable chemicals that pose potential health risks, making their removal from water crucial. MOF membranes can achieve over 84% rejection rates for short-chain PFAS, demonstrating significant effectiveness in water treatment This membrane is enhanced by modifying PA through an interfacial polymerization process, which improves its structure and functionality. The PA-modified MOF membrane features a dense structure and a strong negative surface charge and can remove 11 different types of PFAS, which include both short-chain and long-chain variants. Notably, it achieved over 84% rejection rates for short-chain PFAS, indicating high selectivity. Additionally, the PA-modified MOF membrane exhibits a remarkable water permeance of 21.4 L·m⁻²·h⁻¹·bar⁻, indicating efficient filtration capabilities [124]. The hydrophilicity of short-chain PFAS presents challenges, necessitating innovative approaches like ion-exchange mechanisms to enhance removal efficiency. The hydrophilic nature of short-chain PFAS leads to increased solubility in water. This makes them more challenging to remove from water sources using conventional methods, as they remain dissolved rather than adsorbing onto materials like MOFs. Further research can focus on exploring modifications to existing MOFs or creating new materials that can better interact with these hydrophilic substances [125].
While MOF-based membranes show great promise for PFAS removal, challenges remain, particularly regarding the effective treatment of short-chain variants and the long-term stability of these materials in real-world applications.
Membrane title | contaminant type | Efficiency | Reference |
Cu-TCPP MOF membrane modified by polyamide | PFAS | > 84% | 124 |
MOFs-derived Cu/Carbon membrane | PFOA | 98.22 % | 126 |
CuBTC@PVDF membran | PFAS | 79.64 % | 127 |
ZIF-L/PEI thin film nanocomposite membrane | PFAS | > 95% | 128 |
MIL–101 (Cr)@AC | PFOS | >70 % | 129 |
UiO–66 MOF mixed-matrix membranes Prepared from poly(ethylene-co-vinyl acetate) | PFOA | 100% | 130 |
Pharmaceutical removal
By-products of pharmaceutical industries and industrialized cities and personal care residuals in crowded cities are necessary because they contain toxic components. One of the major concerns about pharmaceuticals is the fact that their effects might be hidden and show themselves after a considerable time or even the next generations, which could bring irreversible damage to human society and its sustainability [131,132]. Great numbers of pharmaceuticals have fluoride groups in their structures like fluoroquinolone, which is one of the most popular groups of antibiotics. Moreover, removing acetaminophen, tetracycline, and diclofenac residuals are the most popular topics in the water and wastewater field. The organic nature of pharmaceuticals and their shape, size, and surface charges are alike to most organic dyes so it is logical to presume that removing them from water resources is quite similar to dyes [133]. Using porous nanomaterials like MOFs to remove this type of pollutant has a history of at least two decades because they can adsorb pharmaceutical residuals without by-products with high efficiency [134].
Membranes, adsorbents, and catalysts are among the popular approaches where MOFs have been used for the remediation of these organic pollutants. MOF-based membranes with two sieving and adsorption mechanism were part of much research in the past few years to purify water from pharmaceuticals, besides they can solve the poor recyclability and complex operational process of MOFs, which is highly desirable [135,136]. Zhao and his colleagues introduced four common MOFs including ZIF–8 and ZIF–67 into the chitosan matrix and remove tetracycline from water. The prepared composites showed a great rejection rate and kept this performance after 10 cycles [137]. Basu and his colleagues fabricated TFC membranes in two different but similar methods where polysulfone (PSF) was used as a support and ZIF–8 was incorporated into PA layers. The optimized membrane indicated a more than 55% retention rate for acetaminophen and high water permeation rate [138]. Huang and his colleagues incorporated poly (sodium methacrylate) (SPA)-grafted UiO–66-NH2 into the polypyrrole selective layer and fabricated thin-film nanocomposite (TFN) membrane for removing antibiotics and polypeptides from water. The resulting TFN membrane revealed more than 99 rejection rate with high permeation rate [139]. Recent studies demonstrate that MOFs can effectively adsorb various pharmaceutical contaminants, showcasing their potential as advanced materials for environmental remediation. MOFs possess a crystalline structure with ultrahigh porosity, allowing for extensive adsorption of pollutants. Its adsorption mechanisms were reported selective. For instance, Cationic Hf(IV)-based MOFs, such as 1’@MeCl, exhibit rapid and selective adsorption of nonsteroidal anti-inflammatory drugs (NSAIDs) including diclofenac, naproxen and ibuprofen, achieving over 99% removal in under 30 seconds from the wastewater environment. Moreover, this 1’@MeCl-anchored membrane was developed successfully to extract diclofenac, naproxen, and ibuprofen from contaminated water [140]. Studies utilizing density functional theory (DFT) reveal insights into the interactions between pharmaceuticals and MOFs, aiding in the design of more effective adsorbents [141, 142]. While MOFs show promise in pharmaceutical removal, challenges remain, such as the need for cost-effective synthesis and scalability for widespread application in wastewater treatment systems.
Membrane title | Drug name | Efficiency | Reference |
CoFe-MOF/TiO2/PVDF | tetracycline | 92.9% | 143 |
TCPP@UiO–66/PAN | diclofenac | over 95 % | 144 |
HKUST–1@PSU membrane | oxytetracycline (OTC), tetracycline (TC), and chlortetracycline (CTC) | 91 %, 91 %, and 99 % | 145 |
UiO–67(Zr)@cyclodextrin-based nanofiber membrane | ibuprofen | 100 % | 146 |
1’@MeCl-anchored cellulose acetate-chitosan membrane | diclofenac, naproxen, and ibuprofen | >99% | 140 |
MIL10-OH/Chitosan embedded membranes | diclofenac sodium and cefixime | 95 % 90 % | 147 |
MIL–100(Fe) modified nanofiber membrane | levofloxacin | 84% | 148 |
CoFe-Layered double hydroxides membrane | tetracycline | 98.5% | 149 |
Conclusion and outlook
Compared to many well-known nanomaterials, MOFs are considered newly developed particles but they found themselves in the market, industry, and research field. By 2013, it had been reported that more than 20,000 MOFs were synthesized and the number of synthesized MOFs surpassed 50,000 in 2021 [150,151]. All of the mentioned data indicate that MOFs are among the most interesting topics in the research fields and there is no obvious limit regarding their applications in a different area. MOFs have a great surface ratio area, they are open to further modification, besides changing linkers, and the shape of MOFs is easily doable, which are highly desirable features for water treatment. However, MOFs have very poor recyclability and this means their real applications in the industry might require a huge amount of time and budget and their operation process is complicated so finding the right one among several thousands of MOFs is arduous and probably unattainable. Therefore, going for compositing is a logical and scientific approach, MOF-based membranes not only have excellent recyclability but also need only pure water and a mildly acidic solution for almost full recovery which is affordable and desirable in the industry.
Moreover, researchers specified the removal mechanism of membranes. Ceramic and polymeric MOF-based membranes have been used for almost all types of pollutants and many of them have already been commercialized. Nevertheless, selecting the right MOF and polymer for fabricating the right composite still need more studies to boost efficiency and reduce the cost. One of the great problems in the MOF-based membrane is poor stability, which needs chronic attention; the problem is inorganic nature of MOFs makes their compatibility with polymeric matrices rather weak. Using core-shell MOFs with organic shell has been used as an alternative way, but different MOFs needs different shells so extrapolating this approach to all MOFs is impossible. Using dual modification or incorporating MOFs into thin-film composites is another popular method; however, the number of MOFs and the extra costs in the dual modification method are still obstacles. It seems, coupling MOF-based membranes with other approaches could be a riveting field, which could open a new window in the wastewater field and solve the complexity of treatment where there are many pollutants or the concentration of pollutants is high. Conversely, developing MOF-based membranes with dual applications like MOFs with photocatalyst or self-cleaning features is getting more attention especially when it comes to organic pollutants like PFAS, phenolic compounds, and organic dyes.
Statements and Declarations
Competing Interests
The author declares no conflicts of interest.
Ethics approval
Not applicable.
Data availability
Not applicable.
Funding
No funding was received to support this work.
Authors’ Information
Matineh Ghomi—Department of Chemistry, Jundi-Shapur University of Technology, Dezful, Iran;