Visual Abstract
Abstract
This study explores the application of zinc oxide nanoparticles in food packaging. Different synthesis methods, including sol-gel, hydrothermal, precipitation, and green synthesis are examined. The unique properties of zinc oxide nanoparticles such as antibacterial, antioxidant, UV absorption, enhanced mechanical strength, and resistance to moisture and oxygen are analyzed in relation to their effect on the physical and chemical properties of nanocomposite films. The role of zinc oxide nanoparticles in improving packaging quality and preventing microbial contamination is discussed. Strategies for incorporating zinc oxide nanoparticles into biopolymers to produce nanocomposite films are also addressed. The study emphasizes the need for further advancements in the stability, scalability, and safety of zinc oxide nanoparticles for industrial and food applications. Ultimately, combining zinc oxide with other materials could lead to the development of smarter and more efficient food packaging solutions.
Introduction
Nanoparticles, due to their extremely small size and unique properties at the microscopic scale have gained significant importance in various scientific and industrial fields in recent years [1]. These properties include high surface-to-volume ratio, specific magnetic, optical, and chemical characteristics, and the ability to interact with molecules and substrates, which enable nanoparticles to be applied in various environmental, medicinal, and industrial processes [2]. Fe3O4 nanoparticles (iron oxide) are widely used in water purification and pollutant removal due to their magnetic properties and ease of separation from aqueous environments [3]. CoFe2O4 nanoparticles (cobalt ferrite) are also effective in catalysis and pollutant removal due to their magnetic properties and high thermal stability [4]. Cu2O nanoparticles (copper oxide) are known for their effectiveness in removing pollutants, including heavy metals and organic contaminants [5]. ZnFe2O4 nanoparticles (zinc ferrite) are utilized in catalysis and environmental processes due to their magnetic characteristics and chemical stability [6]. Additionally, the composite ZnO/Fe3O4 nanoparticles combine the antibacterial properties of ZnO with the magnetic properties of Fe3O4, providing high efficiency in water treatment and pollutant removal [7]. These nanoparticles and their composites have attracted significant attention in various fields due to their ease of recovery and optimal performance in different processes. Therefore, metal oxide nanoparticles, especially zinc oxide (ZnO) nanoparticles have attracted significant attention in various industries due to their unique properties and distinctive capabilities [8–10]. These nanoparticles are utilized in a wide range of applications, including the rubber, electronics, medical, and cosmetic industries, owing to their characteristics such as availability, low cost, simplicity in synthesis methods, and diverse physical and chemical properties [11, 12]. ZnO nanoparticles are particularly recognized for their outstanding features, such as UV absorption, antibacterial, and antifungal properties, making them a material with enormous potential in the food packaging industry [13–15].
In the food industry, ZnO nanoparticles serve as an effective agent in extending the shelf life and maintaining the quality of food products. Due to their antimicrobial properties, these nanoparticles, especially in food packaging are capable of preventing the penetration of contaminants and pathogens into the packaging and preventing the transmission of foodborne diseases to humans [16, 17]. Additionally, ZnO has been approved by the U.S. Food and Drug Administration (FDA) as a “GRAS” (Generally Recognized as Safe) substance [18]. This approval provides consumers and manufacturers with assurance that the use of these nanoparticles in the food industry is safe and entirely risk-free.
In the incorporation of nanoparticles into polymer matrices, two main approaches are commonly used: in-situ and ex-situ methods [19]. In the in-situ approach, nanoparticles are synthesized within the polymer matrix during the polymerization process, resulting in a uniform polymer-nanoparticle composite at the nanoscale. This method typically enhances the mechanical, chemical, and functional properties of the polymer. For instance, in studies like the PAN-Mel@Fe3O4 (Polyacrylonitrile-Melamine) composite, magnetic nanoparticles are directly added to the polymer matrix in the in-situ polymerization process, leading to increased magnetic properties and the ability to adsorb pollutants like cadmium and lead [20]. In contrast, in the ex-situ approach, nanoparticles are synthesized separately and then incorporated into the polymer matrix. Nanoparticles are typically prepared in a separate step before being integrated into the polymer. For example, in studies like the MIL–101 MOF/ZnO/Fe3O4/AG/Chl composite, nanoparticles are added ex-situ to the polymer matrix. In this method, base materials such as MIL–101 MOF (Metal-Organic Framework) and gum arabic (AG) are prepared as the polymer matrix, and then ZnO and Fe3O4 nanoparticles are separately synthesized and added to the hybrid structure. This nanocomposite improves photocatalytic reduction and dye adsorption (such as Erichrome Blue-T) from water, enhancing the photocatalytic system’s efficiency in water purification [21].
Moreover, ZnO nanoparticles have applications in other industries as well. In the concrete industry, these nanoparticles act as a strengthening agent to enhance the resistance and durability of concrete under harsh environmental conditions [22, 23]. In photocatalysis, ZnO is utilized as an active material in the degradation of pollutants and water purification processes [24]. In electronics, these nanoparticles are used as semiconductor materials in the production of sensors, diodes, and other electronic devices [25, 26].
Given the unique properties and capabilities of ZnO nanoparticles in enhancing material properties and industrial processes, these nanoparticles, especially in food packaging have become one of the key and effective materials in maintaining the quality and safety of products. The application of ZnO nanoparticles in food packaging, particularly in preventing the growth of pathogens and extending the shelf life of food products, can be considered as an innovative approach in the food packaging industry [27, 28]. This review will discuss the synthesis processes of ZnO nanoparticles, their physical and chemical properties, and their applications in food packaging.
Synthesis and Characterization of ZnO Nanoparticles
ZnO is widely used in various fields such as catalysis, sensors, photocatalysis, medicine, and renewable energy due to its unique properties, including semiconductor, optical, and antibacterial characteristics [29]. The synthesis of ZnO nanoparticles is crucial for producing materials with specific properties, and it can be achieved through various methods. Each of these methods has its own advantages and disadvantages, and selecting the appropriate method requires consideration of factors such as size, shape, and structural properties of the nanoparticles. Several methods are available for the synthesis of ZnO, and in this study, sol-gel, hydrothermal, and green synthesis methods have been utilized. These methods were chosen due to their ability to precisely control the size and shape of nanoparticles and their potential for producing nanoparticles with specific characteristics. Furthermore, the use of green synthesis as an environmentally friendly method, especially in the production of ZnO with biological and medical properties is of significant importance.
Sol—Gel method
The sol-gel method is one of the most widely used techniques for the synthesis of metal oxide nanoparticles, including ZnO. This method has gained significant attention due to its simplicity, low cost, and the ability to precisely control the physical and chemical properties of the produced materials [30, 31]. In this process, metal precursors are dissolved in a solution to form a gel, which is then converted into nanoparticles through various processing steps. ZnO, as a semiconductor with unique properties has a wide range of applications in areas such as catalysis, sensors, photocatalysis, and medicine [32, 33]. The sol-gel synthesis of ZnO allows for precise control over the size, shape, and structure of the nanoparticles, making it suitable for large-scale production of nanoparticles with desirable characteristics.
Kadhum and et al synthesized ZnO nanoparticles using the sol-gel method[34]. Initially, zinc acetate dihydrate was dissolved in double-distilled water under continuous stirring to form a homogeneous solution. After complete dissolution, absolute alcohol was added slowly to the solution while stirring was maintained. Subsequently, hydrogen peroxide (47%) was introduced dropwise to the solution, and the mixture was stirred using a magnetic stirrer until a nearly clear solution was obtained. This solution was left to stand at room temperature for 24 hours to allow gelation. Following this, the resulting gel was dried at 80°C for several hours to yield a white powder of zinc oxide. The synthesized ZnO was washed several times with double-distilled water to remove any residual byproducts. Finally, the ZnO nanoparticles were dried again at 80°C in a hot air oven to achieve complete conversion and stabilize the final product. At the end, the synthesized ZnO nanoparticles were characterized using various analytical techniques including X-ray diffraction (XRD), scanning electron microscopy (SEM), Fourier-transform infrared spectroscopy (FTIR), and UV-Vis absorption spectroscopy to confirm their structural, morphological, and optical properties.
Hydrothermal Synthesis
The hydrothermal method is one of the most effective techniques for synthesizing ZnO nanoparticles. This method involves chemical reactions in a sealed, high-pressure vessel, known as an autoclave, at elevated temperatures. Unlike traditional solution-based methods, the hydrothermal approach offers several advantages, such as the ability to precisely control particle size, morphology, and crystalline phase without requiring high-energy inputs like calcination [35, 36]. This makes it particularly useful for producing well-defined nanostructures with enhanced optical, electrical, and catalytic properties[37]. One of the key advantages of the hydrothermal method is its versatility in using a wide range of precursors, including both inorganic salts and organometallic compounds [38]. The ability to manipulate reaction conditions, such as temperature, pressure, pH, and reaction time, allows researchers to tailor the properties of the ZnO nanoparticles for specific applications. For instance, by adjusting the pH or introducing surfactants, it is possible to obtain various ZnO morphologies, such as nanorods, nanoflowers, nanospheres, or nanosheets. The hydrothermal process is also considered a green and sustainable approach due to its minimal energy consumption and low environmental impact [39, 40]. The synthesis occurs in an aqueous medium, reducing the need for harmful organic solvents. Additionally, the process can be scaled up for industrial applications, making it suitable for large-scale production of ZnO nanoparticles used in a variety of fields, including photocatalysis, energy storage, sensors, and biomedical devices [41]. Another significant feature of the hydrothermal method is its ability to promote crystal growth under mild conditions [42]. The high-pressure environment inside the autoclave accelerates nucleation and crystal formation, resulting in nanoparticles with high crystallinity and fewer defects. This makes ZnO nanoparticles synthesized by the hydrothermal method highly efficient in applications requiring high surface activity, such as photocatalysis for water purification or antibacterial coatings in medical devices.
Mohan and et al. synthesized ZnO nanoparticles using the hydrothermal method[43]. In this synthesis, zinc nitrate was selected as the source of zinc ions. Initially, a specific amount of zinc nitrate was dissolved in deionized water, ensuring thorough stirring to obtain a homogeneous solution. Sodium hydroxide (NaOH) was then gradually added to the zinc nitrate solution to adjust the pH and create an alkaline environment, which is essential for the formation of ZnO nanoparticles. The slow addition of NaOH resulted in the formation of a white precipitate, indicating the initial formation of a zinc hydroxide precursor. To achieve better control over the nanoparticle size and prevent agglomeration, continuous stirring was maintained during the NaOH addition. Once the precipitation process was complete, the mixture was transferred into a hydrothermal autoclave reactor. The autoclave is a sealed vessel that allows the reaction to proceed under high pressure and elevated temperature conditions, crucial for controlling the crystal growth process. The researchers set the autoclave temperature between approximately 100°C and 125°C and maintained the reaction for several hours, typically ranging from one to five hours, depending on the desired particle characteristics. The temperature and reaction time were critical parameters in this process, as they directly influenced the size and morphology of the resulting nanoparticles. Higher reaction temperatures tended to produce smaller particles with more well-defined crystalline structures. The hydrothermal conditions facilitated the crystallization of the ZnO nanoparticles, resulting in specific morphologies, such as nanoflowers or nanorods. After the hydrothermal reaction was completed, the autoclave was allowed to cool naturally to room temperature. The resulting solid precipitate was then separated from the liquid phase through filtration or centrifugation. The precipitate was thoroughly washed multiple times with deionized water to remove residual ions, unreacted chemicals, and other impurities. This washing step was essential to ensure the purity of the final ZnO product, as the presence of any impurities could affect the physical, chemical, and optical properties of the nanoparticles. Finally, the washed precipitate was dried at a moderate temperature of approximately 60°C to obtain ZnO nanoparticles in powder form. To confirm the successful synthesis and characterize the properties of zinc oxide nanoparticles, various analytical techniques such as FT-IR, Thermogravimetric Analysis (TGA), XRD, Transmission electron microscopy (TEM) and photoluminescence (PL) were used. The XRD patterns confirmed that the synthesized ZnO has a hexagonal wurtzite structure, which is the most thermodynamically stable form of ZnO. The researchers calculated the average crystallite size using the Scherrer equation, which was found to be in the nanoscale range. The TEM images also provided detailed visual evidence of the shapes of the nanoparticles, confirming the presence of nanoflowers and nanorod structures. It was observed that the nanoparticles clustered together, which is a typical feature of ZnO nanostructures synthesized under hydrothermal conditions. Figure 1 shows the hydrothermal synthesis of various nanostructures under different conditions.
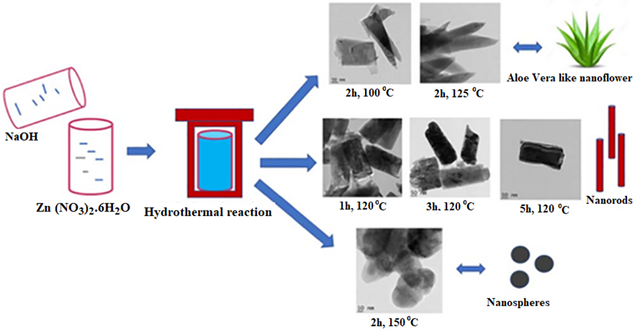
Precipitation Method
One of the synthesis methods for ZnO is the precipitation method, which has gained significant attention due to its simplicity, high efficiency, and low cost. This method is particularly effective in producing ZnO nanoparticles with desirable properties and controlled sizes [44]. The precipitation method, through its simple chemical processes, allows for precise control over the structure and size of the nanoparticles, making it a key option for synthesizing ZnO both on an industrial and research scale [45, 46]. This method is particularly suitable for the production of nanoparticles with narrow size distribution and high surface activity [47].
Qorbani and et al synthesized ZnO nanoparticles using the precipitation method [48]. In this process, zinc nitrate was employed as the zinc source, and potassium hydroxide (KOH) was used as the precipitating agent. Initially, an aqueous solution of zinc nitrate was prepared using deionized water, and a separate solution of potassium hydroxide was also prepared. The KOH solution was then gradually added dropwise to the zinc nitrate solution under vigorous stirring at room temperature to ensure controlled precipitation and prevent the formation of undesired by-products. During this reaction, Zn2+ ions from the zinc nitrate solution reacted with OH⁻ ions from the KOH solution, resulting in the formation of a white zinc hydroxide precipitate. The appearance of this white suspension indicated the successful formation of the precursor. After the reaction was completed, the precipitate was separated from the liquid phase through centrifugation. To ensure the removal of residual ions and impurities, the precipitate was washed several times with distilled water. Finally, it was rinsed with absolute ethanol to enhance the purity of the final product by removing any remaining moisture. The collected precipitate was then subjected to thermal treatment (calcination) in an air atmosphere. The calcination process was conducted at an elevated temperature to convert zinc hydroxide into zinc oxide by eliminating structural water and volatile components. This step also helped to improve the crystallinity of the zinc oxide nanoparticles. The time and temperature of the calcination process were optimized to ensure the formation of nanoscale particles with a narrow size distribution.
To confirm the successful synthesis and characterize the properties of the zinc oxide nanoparticles, they used various analytical techniques such as Uv-Vis, TEM and dynamic light scattering (DLS). The UV-Vis spectrum showed a strong absorption peak at around 370 nm, which was related to the surface plasmon resonance (SPR) of ZnO nanoparticles, confirming the formation of nanoscale ZnO structures (Figure 2a). Furthermore, TEM images showed that the ZnO nanoparticles were well dispersed and had uniform shapes with nanoscale dimensions. This confirmed that the precipitation method employed by the researchers led to the successful production of zinc oxide nanoparticles with controlled size and shape. DLS results showed that the synthesized ZnO nanoparticles exhibited a relatively narrow size distribution with an average size in the nanometer range (Figure 2b). The combination of UV-Vis, TEM and DLS analyses confirmed that the direct precipitation method is a reliable approach for the synthesis of high-purity zinc oxide nanoparticles with desirable structural and optical properties.
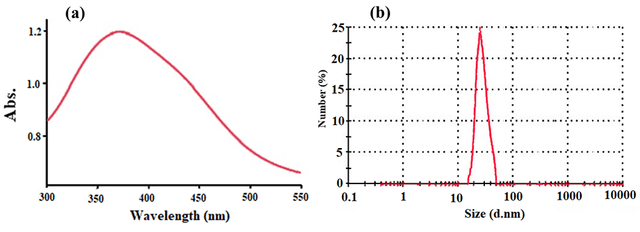
* ZnO: Zinc oxide.
Co-precipitation methods
The co-precipitation method is a simple and cost-effective approach for synthesizing metallic nanoparticles, where metal ions are dissolved in a solution and precipitate as nanoparticles upon the addition of a precipitating agent. Due to its simplicity, low cost, and the ability to control parameters such as pH, temperature, and concentration, this method is widely used for synthesizing nanoparticles with desired size and shape [49, 50]. However, it may lead to issues such as particle agglomeration and lack of uniformity in nanoparticle size. To improve the quality and better control the properties of nanoparticles, various methods are employed, such as the use of a magnetic field to enhance the crystalline structure and increase magnetization, microwave heating to accelerate precipitation and achieve size uniformity, hydrothermal/solvothermal methods to better control temperature and pressure, and ultrasonic treatment to achieve more uniform particle distribution and better control over growth [51]. These techniques help improve the properties of nanoparticles and provide more precise control over their size, shape, and crystalline structure.
Green Synthesis Approach
In recent years, the synthesis of nanoparticles using green methods has received increasing attention [52, 53]. Conventional synthetic methods usually involve the use of toxic and expensive chemicals that can cause serious damage to the environment and human health. For this reason, green methods that use natural resources and non-toxic materials for the synthesis of nanoparticles have been proposed as a suitable and sustainable alternative. In this regard, the use of plant extracts as reducing agents and stabilizers for the synthesis of metallic and semi-metallic nanoparticles has attracted much attention [54, 55]. One of the most important advantages of these methods is their environmental friendliness, in which natural and biological compounds such as plant extracts or other biological compounds are used for the synthesis of nanoparticles instead of using toxic synthetic chemicals[56]. This feature not only reduces the cost of producing nanoparticles but also makes the final products safer for biomedical and environmental applications. In January 2024, researchers used shilajit extract as a natural reducing agent for the synthesis of ZnO nanoparticles [57]. Shilajit is a plant compound known to have antimicrobial and antioxidant properties, and these properties can help in the synthesis of ZnO nanoparticles with unique properties. Furthermore, the synthesis of ZnO nanoparticles is traditionally carried out using chemicals such as sodium hydroxide or sodium borohydride, which in many cases have negative consequences for the environment. Therefore, using a green method with shilajit can be a sustainable, safe and low-cost method for the production of ZnO nanoparticles, which can be very useful in both industrial and biomedical applications.
In this method for synthesizing ZnO, a certain amount of Shilajit powder was added to double-distilled water and the mixture was heated for a specified period at a moderate temperature to extract the bioactive compounds from the Shilajit powder. After cooling the mixture to room temperature, it was filtered to remove solid particles. The obtained extract was stored at low temperature for further use. Next, a solution of zinc acetate was added to the mixture, followed by the dropwise addition of the Shilajit extract. The mixture was stirred for a defined period to ensure uniform mixing. Sodium hydroxide solution was then added to the mixture, and the reaction was allowed to proceed for an extended period at a moderate temperature on a magnetic stirrer to complete the reaction process. After the reaction was completed, the mixture was brought to room temperature and centrifuged at a high speed for a short period. The resulting ZnO nanoparticles were washed to remove any impurities. First, they were rinsed with double-distilled water, followed by isopropanol, and finally dried at a mild temperature for several hours to remove excess moisture and residual solvents.
To confirm the structure of the synthesized ZnO nanoparticles, various techniques were employed. These included UV-Vis spectroscopy for examining optical properties, XRD for determining the crystalline structure, SEM for analyzing morphology and particle size, FT-IR spectroscopy for analyzing functional groups, Energy Dispersive X-ray (EDX) spectroscopy for elemental composition, and DLS for measuring particle size distribution in solution. In the SEM image, it was observed that the ZnO nanoparticles synthesized from the shilajit extract exhibited a morphology resembling nano-flakes, with sizes ranging from 75 to 400 nm (Figure 3). These nanoparticles were predominantly spherical in shape, indicating the unique structural characteristics of the ZnO nanoparticles synthesized through the green method.
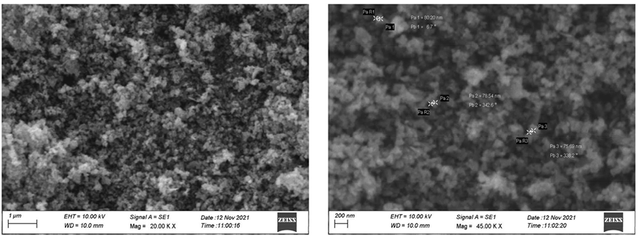
* ZnO: Zinc oxide.
Properties of Zinc Oxide Nanoparticles
Morphological properties
ZnO nanoparticles can exhibit various morphologies depending on the synthesis method used. For example, when the Chemical Vapour Transport (CVT) method is employed for the synthesis of ZnO nanoparticles, tetrapod-like nanostructures are formed. These nanomaterials have four “legs” that automatically align in a plane, with the legs of ZnO nanotetrapods arranged at an angle of 109.5° relative to each other. This unique feature enables ZnO nanotetrapods to perform better in applications such as sensors and catalysts [58, 59]. If different hydrothermal conditions are used for the synthesis of ZnO nanoparticles, a variety of morphologies can be obtained. One of these morphologies is nanowires, which are ZnO nanostructures produced via hydrothermal synthesis. Nanowires are solid, rod-like structures whose thickness or diameter is restricted to tens of nanometers or less (1 nm = 10⁻⁹ m), resembling conventional wires. These nanowires are classified as one-dimensional (1D) materials due to the significant difference between their diameter and length. Furthermore, it has been reported that nanowires exhibit fewer structural defects compared to their bulk counterparts [60–62]. Due to the importance of ZnO nanoparticle morphology, Table 1 has been created to display various ZnO morphologies obtained through different synthesis methods [63–70]. This table outlines the various synthesis techniques for ZnO along with their corresponding morphologies.
Entry | Nanomaterial | Method of Synthesis | Morphology | Ref. |
1 | Nanotubes | Chemical Precipitation | ![]() | 63 |
Entry | Nanomaterial | Method of Synthesis | Morphology | Ref. |
2 | Nanotetrapod | CVT | ![]() | 64 |
3 | Nanosphere | Precipitation | ![]() | 65 |
4 | Nanowires | Hydrothermal | ![]() | 66 |
Entry | Nanomaterial | Method of Synthesis | Morphology | Ref. |
5 | Nanofowers | Hydrothermal | ![]() | 67 |
6 | Nanoplates | Hydrothermal | ![]() | 68 |
7 | Nanorods | Sol–gel | ![]() | 69 |
Entry | Nanomaterial | Method of Synthesis | Morphology | Ref. |
8 | Nanotripods | Hydrothermal | ![]() | 70 |
Structural properties
ZnO nanoparticles typically possess a wurtzite crystal structure, where zinc (Zn) and oxygen (O) atoms are arranged in a symmetrical hexagonal network. This structure gives ZnO notable piezoelectric and pyroelectric properties, which are important in various applications such as sensors and electronic devices. Under certain conditions, ZnO can transform into a cubic zinc blende structure, but this structure is generally more stable at higher temperatures and under specific pressure conditions. Additionally, ZnO can adopt another cubic structure known as rocksalt or halite (sodium chloride) in some conditions, where zinc and oxygen atoms are arranged in a cubic lattice with alternating positions. This structure may temporarily form at very high temperatures or under specific pressures, but in general, the wurtzite structure remains the most stable and common structure of ZnO at room temperature [71, 72]. Figure 4 illustrates the different crystal structures of ZnO.
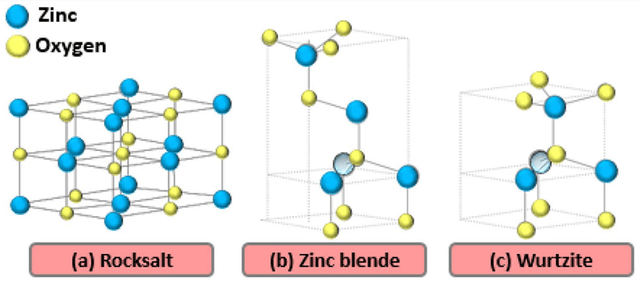
* ZnO: Zinc oxide.
Antimicrobial properties
ZnO is known as a nanoparticle with antimicrobial properties, capable of exhibiting significant antibacterial and antifungal effects [73]. This material attacks the surface of microorganisms by generating reactive oxygen species (ROS) and releasing metal ions, leading to the degradation of the cell wall and ultimately cell death [74]. ZnO has the ability to penetrate the cell membrane and disrupt essential cellular functions, including enzymatic activity, metabolism, and protein synthesis, thereby demonstrating its antimicrobial effects [75].
In their study, Djearamane et al. investigated the antifungal effects of ZnO nanoparticles on C. albicans, and the results revealed the dose-dependent ability of these nanoparticles to inhibit fungal growth [76]. This study showed that ZnO nanoparticles effectively reduced fungal growth and exhibited significant antifungal activity. Furthermore, the results demonstrated that the accumulation of ZnO nanoparticles on the surface of fungal cells led to the destruction of cellular structures and damage to the cell membrane and wall. These changes resulted in severe disruption of cellular function and ultimately cell death. Overall, the findings of this study indicate that ZnO nanoparticles can act as an effective antifungal agent in treating infections caused by Candida albicans. Also, the antifungal mechanism of ZnO nanoparticles generally involves the release of Zn2+ ions from the nanoparticles and the formation ROS, which cause damage to the cell membrane, structural alterations in the cell wall and membrane, and ultimately lead to cell death (Figure 5).
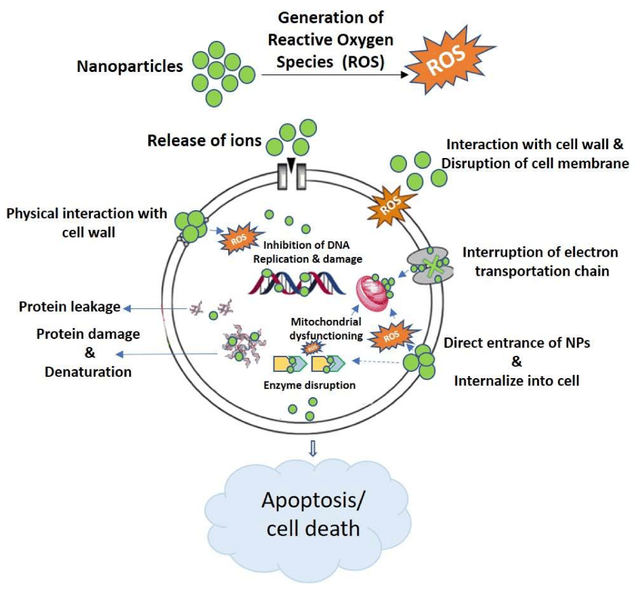
* ZnO: Zinc oxide.
Magnetic properties
In this section, the magnetic properties of ZnO and the impact of doping with magnetic ions in the formation of these properties are discussed. ZnO is naturally a non-magnetic semiconductor; however, by adding magnetic ions such as Mn, Co, and Ni to its crystal lattice, magnetic properties emerge in this material [77, 78]. These ions act as magnetic impurities in the ZnO structure, leading to the uncoupling of electron spins and the formation of magnetic characteristics. One of the key doping processes is the introduction of Mn into ZnO, where the 4s² electrons of manganese transfer to s-p³ bonds in the ZnO structure, resulting in the formation of a tetrahedral structure [79, 80]. This interaction aligns the spins and induces magnetism in ZnO. Additionally, surface defects in ZnO nanocrystals play a significant role in enhancing the magnetic properties. Defects such as oxygen vacancies or zinc substitutions in the ZnO crystal lattice lead to the formation of localized magnetic polarons (BMPs), which align the electron spins and consequently strengthen the magnetic property [81]. In ZnO nanocrystals, which have a high surface-to-volume ratio, these defects have a more pronounced effect on magnetic characteristics. These properties are particularly relevant in spintronic applications, where the spin characteristics of electrons are utilized for data processing and storage. ZnO, through doping with magnetic ions and surface defects, can be recognized as a valuable material in magnetic technologies and spintronics [82, 83].
Figure 6 illustrates the various classifications of semiconductors based on the presence or absence of magnetic ions in their crystal lattices. In Figure 6(a), semiconductors that inherently exhibit magnetic properties or gain these properties through doping with magnetic ions such as Mn or Co are shown. For instance, when Mn is doped into ZnO, it imparts magnetic properties to the material. Figure 6(b) addresses dilute magnetic semiconductors (DMS), in which magnetic properties arise due to the incorporation of magnetic ions into the semiconductor crystal lattice. These properties are particularly observed in materials like Mn-doped ZnO. Finally, in Figure 6(c), non-magnetic semiconductors are depicted, which lack magnetic impurities in their structures and inherently do not possess magnetic properties [84].
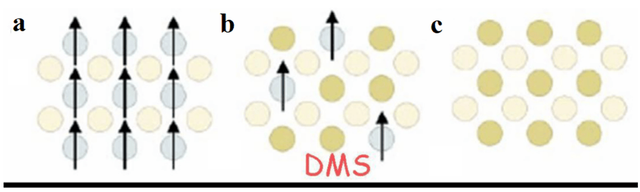
* DMS: Dilute magnetic semiconductors
Thermal properties
ZnO, as a key semiconductor in high-temperature and high-power technologies, exhibits significant thermal properties. Its thermal conductivity is strongly influenced by vibrations and movements within its crystal lattice and is typically limited by phonon scattering [85]. In pure crystals, phonon-phonon scattering plays a central role in heat transfer, but the presence of point defects in the crystal structure of ZnO reduces its thermal conductivity [86]. The thermal conductivity of ZnO varies depending on the quality of the material and the growth method used. For instance, higher thermal conductivity values have been observed in vapor-grown samples and on the polar surfaces of the crystal, highlighting the sensitivity of this property to the crystal structure [87]. Additionally, the thermal expansion coefficient of ZnO, which describes the dimensional changes of the lattice with temperature, demonstrates a predictable response to temperature variations [88]. The wurtzite crystal structure of ZnO plays a crucial role in its thermal characteristics, as the arrangement and interactions of its atoms significantly affect energy transfer[84]. Despite its inherent properties, such as thermal stability and responsiveness to temperature, the presence of defects and the growth processes can substantially alter its thermal performance [89]. These characteristics make ZnO an attractive material for applications such as thermal sensors and high-power electronic devices, as its ability to withstand high temperatures and manage heat is particularly valuable [90].
Role of Zinc Oxide Nanoparticles in Food Packaging
Antimicrobial Mechanisms in Food Packaging
In recent years, the increasing microbial resistance to antibiotics has posed significant challenges to the food industry [91]. Food packaging not only helps preserve quality and extend the shelf life of products but also serves as a protective barrier against microbial contamination. To address this issue, researchers have explored various antimicrobial agents for incorporation into packaging materials. Among these, zinc oxide nanoparticles (ZnO NPs) have gained significant attention due to their potent antibacterial properties and biocompatibility. Krishnamoorthy and et al, recently investigated the antibacterial effect and mechanism of ZnO NPs, highlighting their efficacy against a broad spectrum of pathogenic bacteria [92]. Due to their unique physicochemical properties, ZnO NPs exhibit significant potential in inhibiting bacterial growth and survival. Their antibacterial activity is primarily attributed to multiple mechanisms, including the induction of oxidative stress, disruption of bacterial membrane integrity, inhibition of essential bacterial enzymes, and direct interaction with intracellular components. One of the fundamental antibacterial actions of ZnO NPs is the generation of ROS, such as hydrogen peroxide (H2O2), hydroxyl radicals (OH•), and superoxide ions (O2⁻). The excessive accumulation of these ROS in bacterial cells leads to oxidative stress, resulting in lipid peroxidation, degradation of essential proteins, and DNA damage. Consequently, metabolic processes are severely impaired, ultimately leading to bacterial cell death. In addition to oxidative stress, ZnO NPs interact directly with the phospholipid bilayer of bacterial membranes, triggering lipid peroxidation and increasing membrane permeability. This disruption compromises the structural integrity of the cell, leading to leakage of intracellular components such as proteins, reducing sugars, and DNA into the extracellular environment. The loss of these essential biomolecules results in cytoplasmic destabilization and bacterial lysis. Another crucial antibacterial mechanism of ZnO NPs is their ability to inhibit β-lactamase enzymes, which play a key role in bacterial resistance, particularly in Gram-negative pathogens such as Escherichia coli and Klebsiella pneumoniae. By deactivating these enzymes, ZnO NPs restore bacterial susceptibility to β-lactam antibiotics, including penicillins and cephalosporins, thereby enhancing the efficacy of conventional antimicrobial agents. Furthermore, ZnO NPs can directly interact with intracellular macromolecules such as essential proteins, metabolic enzymes, and nucleic acids, leading to structural and functional disruptions. These nanoparticles interfere with enzymatic activity, disrupt DNA integrity, and impair cellular division, ultimately halting bacterial growth and proliferation. Collectively, these mechanisms render ZnO NPs a highly effective broad-spectrum antibacterial agent with remarkable stability and efficiency. Their incorporation into food packaging materials presents a promising strategy for preventing microbial contamination and extending the shelf life of food products. As research in this field continues to advance, the development of ZnO-based antimicrobial packaging could significantly contribute to improving food safety and reducing foodborne illnesses.
Barrier properties: Resistance to Oxygen, Moisture, and UV Radiation
ZnO nanoparticles are recognized as effective additives for improving the barrier properties of food packaging films. These nanoparticles can enhance resistance to oxygen and moisture penetration, which are crucial features for maintaining food freshness and extending shelf life [93]. When ZnO nanoparticles are uniformly dispersed in the polymer matrix, their high surface-to-volume ratio allows them to act as absorbents, attracting polymer chains to their surface [94]. This interaction reduces the entropy of the polymer chains, and the adsorption interactions lead to a decrease in enthalpy. As a result, the free volume is reduced, and the mobility of the chains is limited [95]. Additionally, due to the hydrophobic nature of ZnO, less moisture is absorbed by the polymer matrix, minimizing the negative effects of moisture [96]. The uniformly dispersed ZnO nanoparticles create tortuous pathways that make it difficult for gases like oxygen to pass through [97]. This characteristic ensures that the nanocomposite is protected from moisture and oxidizing gases like oxygen. Furthermore, ZnO, due to its crystalline structure is capable of absorbing UV rays [98]. When UV rays hit ZnO, it absorbs them and converts the energy into heat, preventing the UV radiation from reaching the intended surface. In this way, ZnO effectively performs both scattering and absorbing of UV rays. In this regard, Channa and et al in 2022 worked on the production of PVA/ZnO nanocomposite films for food packaging [99]. In this study, they used a hydrothermal method to synthesize ZnO nanoparticles. A 15% solution of polyvinyl alcohol (PVA) in distilled water was then prepared, and ZnO nanoparticles were added to it. The solution was stirred for 20 min and then spread onto a PET substrate using a doctor blade. The resulting films were dried at room temperature for 24 h. These films not only acted as barriers to UV and oxygen for food packaging but also prevented moisture penetration. Figure 7 shows the water vapor transmission rate (WVTR) for PVA films and their nanocomposites. With the increase in ZnO content, the WVTR significantly decreased, and the lowest WVTR value was achieved when the nanofiller level reached 0.8%. This indicates that the addition of ZnO improves the film’s ability to resist water vapor, as ZnO creates more tortuous pathways for water vapor to pass through, thereby reducing moisture penetration.
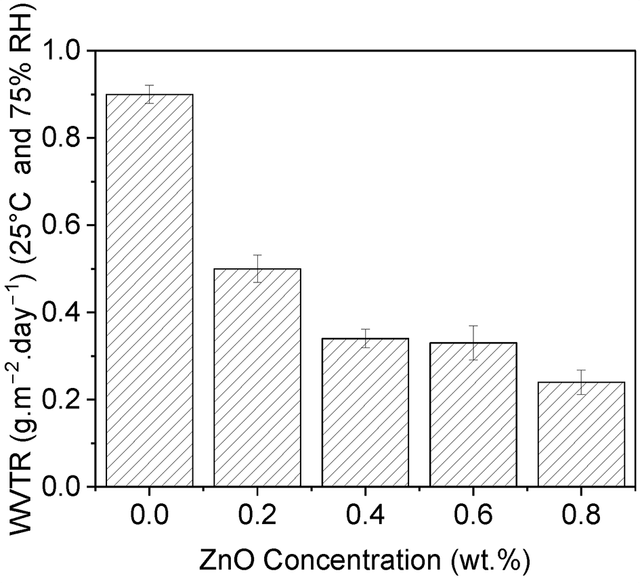
* ZnO: Zinc oxide.
Photocatalytic Degradation of Contaminants
ZnO nanoparticles have garnered significant attention for their photocatalytic properties, making them highly effective in the degradation of environmental contaminants. When exposed to UV light, ZnO nanoparticles generate electron-hole pairs, which initiate redox reactions capable of breaking down organic pollutants in water and air [24]. The photocatalytic activity of ZnO is attributed to its wide bandgap, which allows it to absorb UV radiation and create highly reactive species, such as hydroxyl radicals and superoxide anions [100]. These reactive species can effectively degrade a wide range of organic contaminants, including dyes, pesticides, and industrial waste products [101]. Moreover, ZnO’s high surface area, combined with its low toxicity and stability, enhances its performance as a photocatalyst [102]. However, challenges such as the rapid recombination of electron-hole pairs and limited visible light absorption have been addressed through doping or coupling ZnO with other materials to improve its efficiency under visible light[103]. This makes ZnO nanoparticles a promising candidate for sustainable environmental cleanup, particularly in wastewater treatment and air purification applications [104]. Their ability to degrade harmful substances without generating secondary pollution further highlights their environmental advantages [105]. In food packaging, the photocatalytic properties of ZnO nanoparticles can be utilized to improve the safety and shelf-life of packaged goods. By degrading harmful microorganisms and contaminants that may affect the food, ZnO helps maintain food quality and extend its freshness [106]. Additionally, their ability to absorb UV radiation can prevent the degradation of food products caused by UV exposure, providing an extra layer of protection for perishable items [107].
Strategies for Incorporating ZnO-NPs in Food Packaging
ZnO nanoparticles have attracted significant attention in the food packaging industry due to their remarkable properties, such as antimicrobial, UV-blocking, and antioxidant effects [108]. These nanoparticles can help increase the shelf life and safety of food products by preventing bacterial growth and degrading harmful UV radiation [109]. Their small size and large surface area make them an ideal candidate for incorporation into food packaging materials. Various methods are available for integrating ZnO nanoparticles into food packaging, including blending with polymers, coating, and embedding them in nanocomposites [110]. These approaches offer a promising solution to meet the growing demand for functional and sustainable food packaging materials. This review will discuss several of these methods and applications.
ZnO-Infused Biopolymer Films for Improved Food Packaging
In recent years, there has been an increasing interest in the use of bio-based materials for the production of packaging films and coatings due to their biodegradability and environmental compatibility [111]. One of the widely used bio-based materials in this regard is carboxymethyl cellulose (CMC), which is employed in the production of packaging films and coatings because of its hygroscopic properties, formability, and biodegradability [112]. Gelatin (GEL), due to its biocompatibility and compatibility with food materials is also recognized as a suitable polymer for film and coating production [113]. One of the innovative methods to improve the properties of these films is the incorporation of nanoparticles. Due to their small size and high reactivity, nanoparticles can significantly influence the physical, biological, and chemical properties of polymeric materials. ZnO-Nps are among the nanoparticles that have found extensive applications in various industries, including food packaging and pharmaceuticals, due to their unique antimicrobial and antioxidant properties.
In 2022, researchers synthesized CMC/GEL nanocomposite films containing ZnO nanoparticles and investigated the effect of these nanoparticles on the properties of the films [114]. The aim of this research was to improve the quality and safety of food packaging by adding ZnO nanoparticles to bio-based polymer films. The ZnO nanoparticles were synthesized via a green method using mint leaf extract as a reducing agent. The results showed that the addition of ZnO nanoparticles to CMC/GEL films significantly improved the mechanical, thermal, and antioxidant properties of the films. These films also demonstrated the ability to eliminate DPPH free radicals, which is important for food packaging. In another study, researchers focused on producing antimicrobial bionanocomposite films based on chitosan, polyvinyl alcohol, and silicon dioxide nanoparticles doped with zinc oxide [115]. The primary objective of this study was to investigate the antimicrobial effects of these films and evaluate their physical and mechanical properties, especially for food packaging applications. For this purpose, ZnO-SiO₂ nanocomposite was added in various amounts to the PVA/ Chitosan (CS) matrix, and films with different ZnO-SiO₂ percentages (0.5, 1.0, 3.0, and 5.0%) were produced. The results showed that these films exhibited strong antimicrobial properties against both Gram-positive and Gram-negative bacteria, particularly Staphylococcus aureus and Escherichia coli. These films were also effective against fungal growth and improved the shelf life of packaged bread while reducing foodborne pathogens.
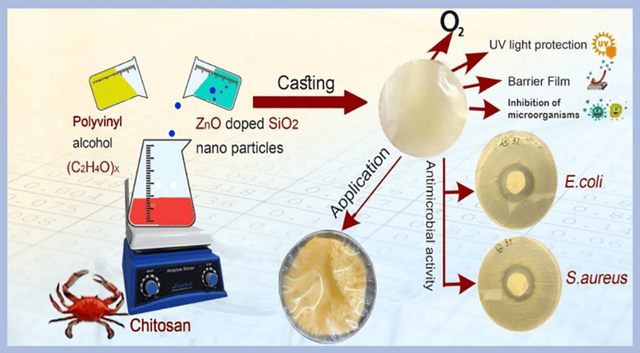
* ZnO: Zinc oxide, CS: Chitosan, PVA: Polyvinyl alcohol
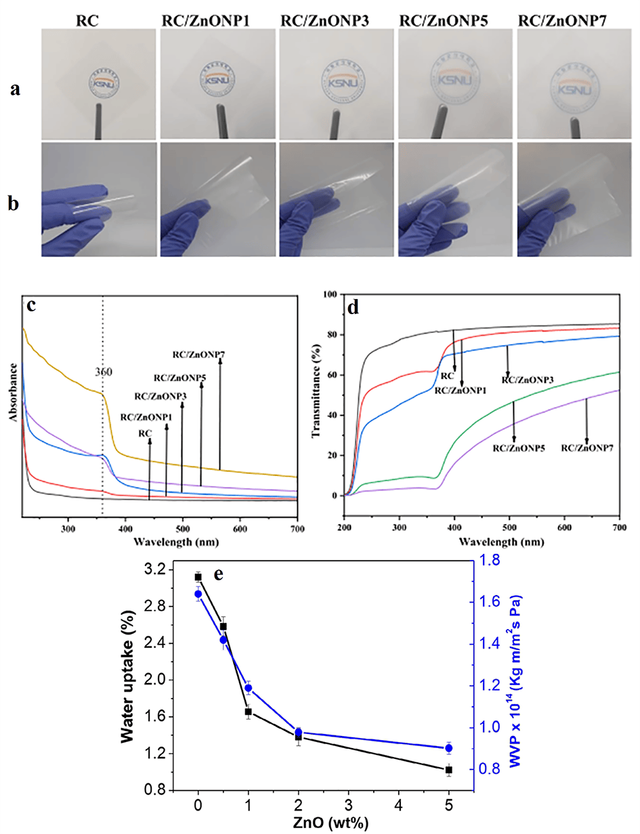
* ZnO: Zinc oxide.
ZnO-Enhanced Biopolymer Coatings for Food Packaging
In today’s world, the packaging industry is increasingly seeking new materials for coatings that, in addition to possessing desirable functional properties have a lower environmental impact [116]. Consequently, there has been a rapid rise in the use of nanocomposites and the incorporation of nanoparticles into polymer matrices for coating applications. Due to their small size and unique properties, nanoparticles can significantly enhance the physical, mechanical, and even antimicrobial properties of packaging coatings, improving both performance and sustainability [117]. In a recent study, Díez-Pascual investigated the effect of ZnO nanoparticles on the various properties of polyhydroxybutyrate hexahydroxy (PHBHHx) biopolymer nanocomposites [118]. The aim of this study was to enhance the physical and mechanical properties, gas and water vapor resistance, and antimicrobial performance of food packaging using PHBHHx/ZnO nanocomposites, offering a sustainable solution for packaging sensitive materials. ZnO nanoparticles were dispersed in an organic solvent, followed by the addition of PHBHHx polymer and ultrasonic treatment for uniform nanoparticle distribution. The mixture was cast into molds and dried to form nanocomposite films. This method improved nanoparticle dispersion and strengthened hydrogen bonding between ZnO and the polymer matrix. ZnO nanoparticles enhanced mechanical properties, gas and water vapor resistance, and reduced water absorption. Additionally, their antimicrobial properties effectively inhibited bacterial growth, improving food protection (Figure 9e).. In another work, recycled cellulose (RC) nanocomposite films were prepared by adding ZnO nanoparticles (ZnONP) to enhance their optical and protective properties for food packaging applications [119]. The results showed that the addition of ZnONP to the RC films reduced their transparency, which was due to the scattering of ZnO particles within the films. However, even with the addition of 3% ZnONP by weight, the films remained semi-transparent, which is suitable for food packaging as it allows for monitoring the freshness of the food. Additionally, incorporating ZnONP into the RC films improved their protective properties against UV radiation, making the films more resistant to UV exposure. This feature enables the films to protect food from UV-induced damage. Furthermore, adding ZnONP to the films decreased their oxygen permeability, which helps maintain the freshness and extend the shelf life of food products. Ultimately, the RC/ZnONP films with 1% and 3% ZnONP concentrations not only showed good UV protection properties but also maintained appropriate transparency and minimized oxygen permeability, making them suitable for food packaging applications. The appearance and flexibility properties of the RC/ZnONP films are shown in Figure 9a-d. As the concentration of ZnONP increased, the transparency of the films decreased, which was due to light scattering by the ZnO particles and UV light absorption at wavelengths below 360 nm.
Hybrid Nanoparticles for Enhanced Food Packaging
Hybrid nanoparticles, as a combination of two or more different materials, exhibit unique properties that provide better performance compared to simple nanoparticles [120]. These nanoparticles have gained particular attention in the field of food packaging, as they can enhance various properties such as antioxidant, antibacterial, and moisture and oxygen barrier capabilities [13]. For example, the combination of silica-silver nanoparticles (SiO2-Ag) or titanium dioxide-silver nanoparticles (TiO2-Ag) is used to create antibacterial films in food packaging, which extend the shelf life of products [121, 122].
In 2022, Gasti and et al. conducted research aimed at improving the antibacterial, antioxidant, and barrier properties of food packaging films by designing and synthesizing Chitosan-Zinc Oxide-Clove Essential Oil (CS-ZnO@CEO) hybrid nanoparticles [123]. The main goal of this study was to enhance the characteristics of nanocomposite films for food packaging applications, thereby increasing the shelf life of food products and preventing microbial growth and oxidation. In this approach, the hybrid nanoparticles were synthesized using the sol-gel method, where chitosan acted as the base polymer for stabilizing zinc oxide and clove essential oil. These nanoparticles were then uniformly dispersed in a chitosan-pullulan matrix to achieve the desired properties in the films.
The properties derived from zinc oxide included its antibacterial effect, improved mechanical properties of the films, and enhanced UV-blocking capabilities. Additionally, zinc oxide, due to its crystalline structure, helped create a denser network in the films, improving both moisture and oxygen barrier properties. Ultimately, the incorporation of these nanoparticles into the films resulted in materials that exhibited strong antioxidant and antibacterial properties, preventing the growth of bacteria such as E. coli and P. aeruginosa. Compared to conventional films, these nanocomposite films significantly extended the shelf life of chicken meat by up to 5 days.
Conclusion and Future Perspectives
ZnO nanoparticles have significant potential to enhance food packaging properties due to their unique features, such as antibacterial, antioxidant properties, and resistance to UV radiation. These nanoparticles can improve the physical and chemical properties of packaging films, extending the shelf life of food products and preventing microbial contamination. The use of these materials in packaging not only enhances performance but also presents a sustainable and environmentally friendly solution. However, further research is needed to assess the scalability and safety of these materials in industrial and food applications. In the future, the combined use of ZnO nanoparticles with other materials could lead to the development of smarter and more efficient packaging solutions. This technology has the potential to be widely applied across various industries to preserve food quality and ensure safety.
Statements and Declarations
Authors' contributions
Writing—Original Draft Preparation; A. J. Review & Editing; N. B. All authors have read and agreed to the published version of the manuscript.
Competing Interests
The author declares no competing interest.
Ethics approval
Not applicable.
Data availability
Not applicable.
Funding
This paper received no external funding.
Authors’ Information
Abolfazl Jahani—Polymer Chemistry Research Laboratory, Faculty of Chemistry and Petroleum Science, Shahid Beheshti University, Tehran, Iran;
Nazila Biglari—School of Biological Sciences, Universiti Sains Malaysia, Penang, Malaysia;