Visual Abstract
Abstract
Regarding water, this fabulous molecule is not just what humans drink to survive but also the source of life for all planets and creatures across the universe. Therefore, water is an invaluable and irreplaceable resource, and the quality of this precious liquid is crucial for the sustainability of society and public health. Regrettably, with the increase in the human population, water pollution is also rising because almost all human activities can culminate in the contamination of the environment, including water. Although abolishing old approaches in favour of eco-friendly ones is catching more eyes, water pollution is still a vital issue in industrialized and developing countries. Finding a proper material to purify most contamination is essential to water remediation. Nowadays, conducting polymer-based composites (CPCs) are gaining more attention due to their unique features like long life, durability, processability, lightweight, high environmental stability, and tunability. Within this review, we will briefly discuss what conductive polymers (CPs) are, and then we will scrutinize the usage of CPCs for the remediation of various pollutants from water. The proposed mechanism of the purification process and the most recent fabrication methods of these new generations of composites will be discussed.
Introduction
Water is a familiar word with only five letters, but it is enough to define the world. Since the dawn, humans have been curious creatures who want to explore their surroundings and find what lies behind them. Discovering Australia, Antarctica, and America was the outcome of this curiosity. However, humans are exploring a more immense and limitless space called the universe. Every culture and nation worldwide has its own stories and anecdotes about sky and space, and this vast area has been a mystery since primal humans. Thanks to new technologies, space probes, and rovers, humans found humankind now found himself on Mars and the moon, but there is much ahead.
Nevertheless, what do humans seek in the sky? What are the key features? One of the most vital ones is water. Although this molecule is colorless and odorless, it can give life, color, taste, and odor to every creature [1,2]. Therefore, searching for water on other planets and galaxies seems logical, and most researchers believe that water means extra-terrestrial life [3]. If water is that precious all over the cosmos, what is its value on the blue planet? Although water covers most of Earth’s surface, drinkable and untainted water is scarce [4,5]. The studies predicted that by 2050, half of the human population would face severe problems accessing clean water. While finding new water sources is almost impossible, protecting current sources is vital [6,7]. Most of humankind’s activities could contaminate water, like eating meat for dinner [8]. It is important to pay close attention to heavy metal pollution, pesticides, dyes, and pharmaceutical residues due to their stability, toxicity, and potential to make drinking water and aquatic life unsafe at trace levels. There is a potential for irreversible environmental consequences from pollutants that persist in the environment and accumulate in the body. Heavy metal levels in soil have increased due to various human activities, including mining, smelting, and agriculture. Aside from heavy metals, heavy metals also produce potent carcinogens. Mina Mata disease and poisoning can occur when drinking water is contaminated with Mercury and arsenic. Animals and plants use heavy metals for various biological and chemical functions. In addition to participating in redox reactions, essential heavy metals also directly regulate enzyme activity.
The environmental threat of emerging organic contaminants (EOCs) has increased significantly over the past few decades. Pharmaceuticals and personal care products (PPCPs) are one of the first sources of water pollution, and other sources include pharmaceuticals, hormones, pesticides, plasticizers, industrial additives, surfactants, and hospital waste. The development of organophosphate pesticides (OPPs) during World War II (WWII) has generated extensive research. As a result of the ban on organochloride pesticides in the 1970s, insecticides and nerve agents have become widely used in agriculture. Paralyzing and eventually killing insects they cause them to become paralyzed. It has been demonstrated that organic dyes are mutagenic, toxic, and carcinogenic due to various toxins, carcinogens, and mutagens. An ecosystem’s microbiome can be disrupted, antibiotic-resistant bacteria could develop, and an organism’s microbiome may be affected by pharmaceutical residues.
As a result of the release of antibiotics, the environment is also exposed to metastable cyclic functional moieties, such as quinolones and morpholines. Although contamination prevention is feasible, cleaning the polluted water might be among the best ways to protect life support. Purifying water has a long history, maybe as long as the first civilization. Using boiled water might be the first purified water usage, but happily, today, there are many reliable and more effective ways [4,9]. During the past decades, activated carbon, activated sludge, photocatalyst, gravimetric method, membrane, filters, electrochemical, adsorbents, and many other approaches were combined to purify the tainted water [10–13]. Each one of them can offer some privileges; however, none of them is free of drawbacks. Furthermore, that is when science can overcome the disadvantages and advance technology.
On top of this, there are different approaches to water treatment; there is also a wide range of materials for water purification. Among new generations of materials, metal-organic framework-based (MOF-based) [14], covalent-organic framework (COF-based) [15], graphene oxide-based (GO-based) [16], nanocellulose-based [17], biochar-based [18,19], conductive polymer-based materials [20] are gaining more attention these days, and each can offer some merits, so choosing the right material is a significant concern. Generally, cost, time, labor, handling, accessibility, and facility are the key considerations for any scientific and industrial methods. Regarding water purification, the type of pollutants and their concentration play an essential role [21].
Polymer is a familiar term, but its world is vast, limitless, and perhaps unknown. This riveting branch of soft matters revolutionized science and, as a result, human life [22]. The application of polymers in water treatment has been recognized for decades, and they have been used for purification purposes solely or along with other materials (mostly known as hybrid systems) [23,24]. Nanotechnology marching at the staggering space and using nanomaterials in wastewater treatment is no exception. However, reaching the efficiency of hybrid systems is arduous, even for the best-fabricated nanomaterials. Polymers can connect all components and play as an anchor for nanomaterials, besides their other fortes like accessibility and processability [25]. Conductive polymers (CPs) are fascinating and have found their place in many applications, including electronics, electromagnetic interference, batteries, water remediation, and other fields [26].
CPs are structures with extended π-conjugated. These polymers are lightweight, durable, tunable, stable, and open to further modification [27]. Among them, polythiophene (PTh) [28], polypyrrole (PPy) [29], polyaniline (PANI) [30], and their derivatives reeled in more eyes and monopolized most research for themselves. Regarding water and wastewater treatment, PANI is the most extinguished one because it is eco-friendly, has facile availability, has high stability against solvents, and has excellent removal capacity [30]. In addition, the oxidation of PANI in different forms could culminate in various derivatives with other applications. Therefore, PANI is a worthy candidate for treating water with complex or multiple pollutants, and the truth is that most of the time, pollution has more than one source [31].
Although it may be a strange comparison, similar to humans, different materials need each other, and they can perform much better in appropriate combinations. Moreover, composites are its embodiment. For instance, polymers alongside nanomaterials (NMs) can surpass the efficiency of NMs and polymers when used separately [32]. Therefore, this proved that efficiency is why composites are usable and available in almost every aspect of modern life. Conversely, conductive polymer-based composites have been proven a potent material for aquatic remediation, and more than 2400 research papers on this particular topic until 2023 (based on the Scopus database) are solid proof for this claim. One of the unique features of this composite type is that there is no chemical reaction or binding between fillers and polymers; instead, they reside in the leading chains of polymers [33]. We will discuss and scrutinize conductive polymer-based composites in the following pages, but laconically, they have purified almost all kinds of pollutants using different treatment methods.
Conductive polymers
Introduction
Organic polymers that conduct electricity are conductive or intrinsically conductive (ICPs). These polymers may possess metallic conductively, or they can be like semiconductors. Processability is their remarkable advantage over most materials with the same ability (conduct electricity) [34]. The other aspect is that most CPs cannot reveal the same mechanical stability as other well-known polymers, and almost none are thermoplastic. Although many methods have been developed over the past decades, dispersion has mainly been used for ICPs processability [35]. Hence, the organic nature of these polymers, alongside advanced dispersion techniques, provides the possibility of fine-tuned materials.
Structure of the main chain | With no heteroatom | Contain heteroatom | |
S-containing | N-containing | ||
Containing aromatic cycles |
|
|
|
Containing double bonds | poly(acetylene) (PAC) | ||
Containing both aromatic cycles and double bonds | Poly(p-phenylene vinylene) (PPV) |
History
Even though there is no certainty in the first usage of CPs, their first description went back to the 19th century when Henry Letheby noticed the difference between two forms of aniline while working on an electrochemical reaction. However, the first reliable reports back to the 70s and 80s were from numerous researchers who discovered that, despite previous beliefs, some polymers could be highly conductive and even play as electrodes. Before this research, all polymers were considered insulators [36]. PPy, oxidized polyacetylenes, and PANI were among the first polymers to prove their conductivity. Professor Shirakawa and his colleagues won the Nobel Prize 2000 in chemistry “for the discovery and development of CPs.”
Types
There are numerous ways to sort CPs, but PANI, polyindole, poly(acetylene) (PAC), and PPy and their Co-polymers are the most common CPs, and all have one similar feature; they have linear backbone. It is worth mentioning that two new polymers got much attention in the recent decade: 1) poly(3-alkyl thiophenes), which are the staple part of transistors and solar cells, and 2) poly(p-phenylene vinylene) (PPV) with massive usage in electroluminescent applications [37]. In Table 1, CPs were sorted based on the structure of their main chain.
Synthesis
There is a variety of approaches for synthesizing CPs. However, they are not the topics of this review, and a brief explanation was brought for an overview. Oxidative coupling is the primary method of synthesizing CPs, and some monocyclic precursors can be used. However, the low solubility of these monomers is a great challenge, and even today, some researchers are introducing novel approaches to triumph over this obstacle. Among all proposed approaches, adding solubilizing functional groups and surfactant stabilizers has been used more frequently [38]. Nevertheless, the low molecular weight of CPs remains a challenge in most cases.
Regardless of the low solubility of monomers, two main methods for CPs are chemical reaction and electro (co) polymerization. In the former method, monomers should be placed under different conditions, like the catalyst, light exposure, heating, and pressing, and the result would be a carbon-carbon bond between monomers. High yield is the most apparent feature of chemical synthesis, although impurities and uncontrolled sub-reactions are this method’s disadvantages. Inserting three different electrodes (working electrode, counter electrode, and reference electrode) into the solution is the mechanism of electro (co) polymerization. The crucial factor in this method is how voltage is applied, where constant or cyclic voltage can drastically shift the final properties of CPs, even though the basis of both approaches would be a redox reaction. Although this novel approach solved the impurity problems of chemical synthesis, the main problem is that only a few products can be provided [39–41].
Features
What is unique about CPs that makes them able to conduct electricity? The usual answer is microstructure. Valence electrons in conventional polymers like polyether sulfone are bound in sigma-bonding electrons. Therefore, these SP3 hybridized bonds can reflect poor mobility and, as a result, low electrical conductivity. Regarding CPs, their backbones are full of continuous SP2 hybridized centers. Pz orbitals are orthogonal toward the other three sigma bonds, critical for more mobility and higher electrical conductivity. After all, a set of delocalized orbitals is the key feature for all conjugated materials [42–44].
The features of CPs are a vast subject worth several reviews, so what can make CPs conductors, semiconductors, or insulators is off-topic for this review. It is worth mentioning that the complexity of CPs is still a riddle, and the relationship between morphology, conductivity, main chain structure, and pending groups is vague.
Applications
Considering that the organic nature of CPs is a remarkable advantage in the first place, the fact that they also have electrical conductivity reveals their unique applications. In recent years, the usage of these fabulous materials in batteries [45], displays [46], solar cells [47], electromagnetic interference (EMI) shielding, supercapacitors [48], sensors [49], actuators [50], diodes [51], and electronic devices [52] has been reported continuously. Besides, areas like water-treatment purposes are a new field for CPs to shine through [53]. Generally, low cost, processability, and physical/chemical stability are common attributes in all CPs. However, the reproducible and stable dispersion of PPy and PANI make them staple parts of many commercial and industrial applications. The other remarkable CPs is polyindole, which has excellent thermal stability, slow degradation, and, most importantly, excellent redox activity [32,34,52]. A summary of conductive polymer application was brought in Figure 1A. In addition, conductive polymer hydrogels are a new generation of hydrogels. They are already being commercialized in various fields, such as separation membranes, adhesive coatings, drug delivery, and many others, as shown in Figure 1B.
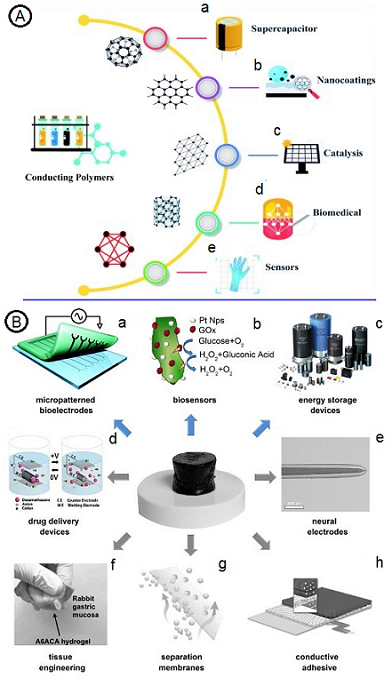
As mentioned in the previous section, one of the most significant limitations of CPs is that they are insoluble, and dispersion is the only way to use them. Moreover, moisture in the air can affect the charged backbone of ICPs and cause unwanted instability. Eventually, optimizing these polymers is arduous because researchers need to learn more about the relationship between the conductivity, structure, and morphology of CPs [20].
Water treatment via conductive polymer-based composites
Introduction
There are various advantages to using composites/nanocomposites (NCs) rather than polymers regarding water treatment. For example, composites mostly have better chemical, mechanical, and thermal stability, higher removal capacity, higher reproducibility, and better selective recognition of pollutants like dyes, heavy metals, and oils. Furthermore, some merits of most composites are low cost, environmental stability, facile preparation, and more active sites [56].
Conductive polymer composites (CPCs) are polymer-based materials with conductive fillers. Generally, CPCs synthesizing and fabrication methods are close to other composites with only two distinct differences: the CPs are primarily insoluble, so dispersion is the primary approach, and conductive fillers are mostly more expensive than other fillers. Evaluating and discussing the features of CPCs is not the subject of this review. However, it could be said that insolubility in water, non-toxicity, and regeneration are the benefits of these NCs [57]. Besides, CPC’s chemical stability and porosity are usually higher than those of other conventional composites. PPy, PANI, its derivatives, and PTh have been used drastically in CPCs and CPs-based NCs [57]. As mentioned above, CPs have found their way through many fields, but what makes them an appropriate choice for fabricating composites and using them in water and wastewater treatment? The answer to this question is not that easy because the source of pollution, the conductive fillers of composites, and the types of CPs are so different. However, one of the best general answers could be the presence of heteroatoms in most conductive polymers [58]. After all, heteroatoms are active sites to adsorb pollutants. Considering this factor, CPs with N atoms have more removal capacity than S atoms. Therefore, the adsorption capacity of PTh is much lower than that of PPy. Using CPCs in water treatment can be sorted into three general types: 1) membranes, 2) photodegradation, and 3) adsorbents [59]. An illustration of using CPCs in water treatment and the proposed mechanism was brought in Figure 2.
CPC as adsorbents
Adsorbents are one of the best methods for water remediation because of their high-speed rate, clean process, free residual reaction, facile operation, and low cost [60–62]. CPCs as adsorbents are the most abundant techniques compared to membranes and photodegrading [63]. Several combinations were reported for the compositing process of CPs, including other polymers, inorganic-based materials, organic-based materials, carbon-based materials, and clay minerals, which is solid proof for the popularity of this kind of composites among researchers.
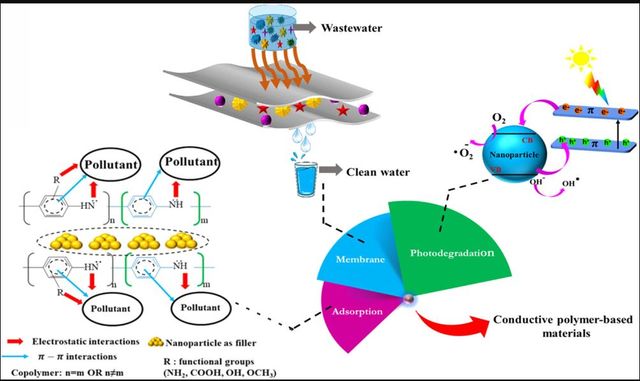
Adsorbents type | Pollutant type | Capacity of adsorbent (mg/g) | Released year | Reference |
PANI/Jute fiber | Cr3+ and Cr6+ | 140 for Cr3+/150 for Cr4+ | 2019 | [67] |
PANI/GO@tea waste | Bromide | 26.8 | 2022 | [68] |
PANI/carbon nanotubes | nitrite | - | 2022 | [69] |
PANI/cellulose | Heavy metal/dye | - | 2022 | [70] |
Ppy@PANI@FBC | Cr6+ | 203.7 | 2022 | [71] |
MSP-PANI | Lead ions | 163.3 | 2022 | [72] |
RmGO/PANI | Moxifloxacin | 47.7 | 2022 | [73] |
PANI/PVA/SA | Cr6+ | 83.1 | 2022 | [74] |
PANI@ Kieselguhr | Bisphenol A | 47.8 | 2022 | [75] |
PANI/Bi2O3 | 4-nitrophenol | - | 2022 | [76] |
MXene/PANI/PPy | salt | 39.62 | 2021 | [77] |
PANI/MnO2 | Pb2+ | - | 2021 | [78] |
PANI-based adsorbents
On the other hand, PANI-based composites (and their close derivatives) caught much attention to themselves as excellent components for adsorbents. The structures of PANI derivatives are so close to each other, and most are different based on the pendant functional groups [64]. Considering amine groups of PANI, these pendant groups can be located in ortho, meta, or para positions. Although PANI has shown poor solubility in most organic solvents, like N-methyl pyrrolidone (NMP), dimethyl sulfoxide (DMSO), and N, N-dimethylformamide (DMF), its derivatives mostly have much better solubility in these solvents; however, better solubility mostly can sacrifice conductivity, and there is a “trade-off” relationship between these two features [65]. The acidic solution, peroxy initiator, and corresponding monomers are required to synthesize PANI derivatives. The PANI composites are excellent adsorbents because this polymer possesses many amine and imine groups in its backbone; however, PANI derivatives with more functional groups can augment the removal capacity of composites. Therefore, removing different pollutants from wastewater via these adsorbents allocated a significant proportion of recent articles about conductive polymer-based composites to themselves [66]. Several recent articles about adsorbent CPCs for removing pollutants were brought in Table 2.
PANI derivatives-based adsorbents
Poly(ortho-phenylenediamine) or PoPDA, poly(meta-phenylenediamine) or PmPDA, and poly(para-phenylenediamine) or PpPDA are three well-known derivatives of PANI, which have been used for years as adsorbents for a variety of pollutions [79]. The Scanning electron microscope (SEM) and Transmission electron microscopy (TEM) images of these derivatives are shown in Figure 3.
Polymerization of ortho-phenylenediamine (oPDA) via ammonium persulfate (APS) can lead to the synthetization of PoPDA [81]. The same approaches were reported for synthesizing PmPDA and PpPDA [82,83]. The adsorption capacity of these derivatives can be affected by numerous factors, like the contact time with pollutants, the pH of the solution, the concentration, and the temperature of the solution. Besides, the pollutant type also dramatically impacts PANI derivatives’ efficiency and removal capacity (also true about PANI). The other major factor is the molar ratio of oxidant/monomer, where a higher molar monomer ratio can culminate in higher removal capacity (a molar ratio of 1:1 oxidant/ratio showed the best result) [84,85]. One possible mechanism based on this behavior could be redox reaction and chelation between imine/amine groups of PANI derivatives and ions. To scrutinize the redox reaction theory, Li and his colleagues tested the removal efficiency of PoPDA while Ag+ and Hg2+ ions were the pollutants. The results revealed a preference for silver ions, another proof of the redox reaction theory [86]. An illustration of the proposed mechanism is shown in Figure 4, where complexation between PoPDA and lead ions can be observed.
As shown in Figure 3, PANI derivatives have many shapes and structures like other CPs, including hollow microsphere, hollow and solid sub-microsphere, micro/nanoparticles, nanorods, nanobelts, and others. Furthermore, it is proven that this shape has some degree of effect on the removal capacity of pollutants. Until now, water with dyes, oils, heavy metals, salts, and organic pollutants pollution was purified with PANI derivatives with high efficiency [79,82,86]. As can be seen in Table 3, some recent articles about these types of adsorbents were gathered.
Adsorbents type | Pollutant type | Capacity of adsorbent (mg/g) | Released year | Reference |
Fe3O4@PpPDA | Mn7+ | 148.3 | 2022 | [87] |
r-GO@PpPDA | Methylene Blue | - | 2021 | [88] |
Ligand-based PpPDA | Phosphate | 69 | 2021 | [89] |
Pectin/PmPDA | Pb2+ | 390.9 | 2021 | [90] |
Zif–67/PmPDA | Cr6+ | 240.4 | 2021 | [91] |
Fe3O4@PmPDA(Z) | Cr6+ | 371.7 | 2021 | [92] |
ZnO@PmPDA | Pb2+ | 77.5 | 2022 | [93] |
Carbon dots@PoPDA | Pb2+/Cd2+ | - | 2021 | [94] |
CMC@PoPDA | Congo Red | 524.6 | 2022 | [95] |
CHCF-poly-AA-co-OPD | Cesium ions | - | 2022 | [96] |
PPy-based adsorbent
PPy is one of the commercial CPs used in many fields, such as batteries, solar cells, capacitors, adhesive coatings, and others. Nevertheless, their usage as an adsorbent is not as common as PANI; these polymers can be coupled with almost all materials to provide stable, cheap, lightweight, and reproducible composites as adsorbents. In addition, their facile oxidative polymerization is a unique privilege. In-situ growth of this polymer on other surfaces is one of the promising approaches that reeled in so much attention to itself. Surfaces like rare element salts are in the special spotlight for this matter. There are many reports that PPy-based adsorbents can be more effective as composites than PPy polymers because more active sites will be available in the composite form [97–99].
Fabricating a composite where in-situ growth of PPy on cerium phosphate adsorbed Cr6+ was investigated under the Langmuir isotherm model. The results revealed that the best results can be achieved at room temperature (r.t) and acidic solution [100]. Conversely, The SEM and TEM images indicated that this approach could alter composite morphology compared to bare PPy, as revealed in Figure 5.
Removing heavy metals
With the rising human population and several industries, water pollution with heavy metals is getting more serious. Many industries like batteries, mining, automobiles, tanneries, and others can culminate in the leakage of heavy metals into water supplies or rivers [101]. Their toxicity and mobility in water resources and ecosystems are significant concerns for sanitation. Therefore, what are heavy metals? Metals with an atomic weight between 63.5–200.5 nm should be considered heavy metals [102]. Almost all heavy metals are stable and non-degradable in the environment. Hence, removing them from the aquatic system is essential. Removing mercury, chromium, lead, copper, arsenic, cobalt, zinc, nickel, and manganese is among the hottest topics in water treatment, mainly because they are the primary pollutants of factories and industries [19,103]. Although some iota dosage of some mentioned metals is necessary for the human body, storing these metals can cause irreversible damage to the body. For example, Hg ions can harm the kidneys, while arsenic is harmful to the lungs and can expedite most cancers, particularly skin cancers [60,104].
On the other hand, copper can cause brain damage and even death. At the same time, an exorbitant amount of cobalt and nickel is detrimental to kidneys, and severe vomiting is another side effect. Moreover, most double-positive metallic ions are among serious water pollutants. Furthermore, the recovery of heavy metals or at least their oxides species is not a commonplace matter. After the removal step, combustion is one of the most desired approaches; therefore, burnable adsorbents are the favorite [105,106].
Remediation of water from hexavalent chromium is one of the major concerns of the twenty-first century. This metal ion is more toxic than most heavy metals and is a staple of many factories’ wastes. To give a proper comparison, the toxicity of Cr6+ is more than 450 times that of Cr3+, so removing this particle has drawn much attention. Adsorbents with abundant functional groups are one of the best ways to treat water supplies with hexavalent chromium contamination [105,106]. Among conductive polymer-based composites, PANI is one of the best choices because it possesses many amine and imine groups. Moreover, its derivatives and related co-polymers have been used on this topic for years. As mentioned earlier, the pH solution, dosage of adsorbents, the temperature of the solution, and other factors directly affect composites’ removal capacity, where the pollution’s type and concentration are influential factors, too [107]. The removal of Cr6+ via PANI-based adsorbents was tested under different pH, and it was found that the best range is 2–4 (acidic solution), but why? Cr ions have many shapes and structures in the aquatic solution, and the significant shape heavily depends on the solution’s pH. For example, in the mildly acidic solution, the main form is CrO4- and the HCrO4- and Cr2O72- were dominant forms under a more severe acidic solution. In a highly acidic solution with pH<2, there is a shift in the chromium ions, and the Cr3+ form is the main structure, so this condition cannot benefit the removal process. The other aspect of the Cr6+ removal process is the activity of amine/imine groups of PANI, which can be investigated by zeta potential [107,108]. As shown in Figure 6A, PANI’s zeta potential peaked around pH 2–3 [109], and this range is the best condition for the complexation between the Cr2O72- and amine /imine groups of PANI. As a result, the proposed mechanism for hexavalent chromium removal can be seen in Figure 6B. On the contrary, removing Cr6+ in the alkali solution is very low because the dominant form in this solution is Cr(OH)3, and groups like amine groups cannot function efficiently [110,111]. In addition, PPy and its derivatives have also been used as adsorbent composites for removing Cr6+, in which NH- groups were responsible for the adsorption. Removing Cr3+ via PANI or PPy needs quite a different situation, and alkali solutions are the best because there is a repulsion between the positive charges of this heavy metal and amine groups [112,113].
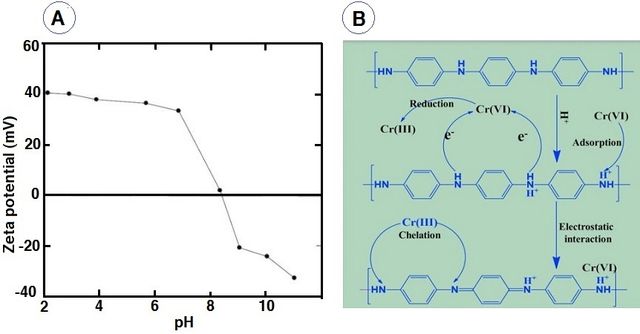
Another heavy metal that requires special attention is mercury ion (Hg2+). Based on the maximum contamination level (MCL) set by the American Environmental Protection Agency (EPA), any amount more than 0.002 mg/L of mercury in the water is undrinkable. Therefore, remediation of water from this heavy metal is necessary and urgent. Like most heavy metals, functional groups like hydroxyl, amine, imine, and phenol are necessary to augment the removal efficiency [115,116]. Therefore, PANI-based adsorbents (and their derivatives) are desirable materials for this purpose, and they have been used in various composites to boost the removal process. Two key factors to boost mercury removal are ionic strength and pH of the solution, although the temperature of the solution and dosage of adsorbents are also effective [117]. Based on the reported literature, higher ionic strength can boost adsorption, but there is a limitation (Figure 7A).
On the other hand, the solution’s pH can change the mercury formation in the water. As seen in Figure 7B, the remediation process of Hg-polluted water in the severe acidic solution is relatively poor, and the best efficiency could be obtained around pH = 4. The dominant form of mercury in the different pH can explain this phenomenon, and as it is clear from Figure 7C, Hg (OH)2 is the main form around pH = 4 [117]. Some explanations were given for the poor adsorption of mercury in severely acidic solutions, including competition between Hg2+and H+, the repulsion force between amine/imine/hydroxyl groups and Hg2+, and semi-stable/low small hydrate size of Hg2+ in pH below 2 [115,116]. Using other conductive polymer-based composite adsorbents, like PPy, PTh, and their derivatives, might be helpful, too.
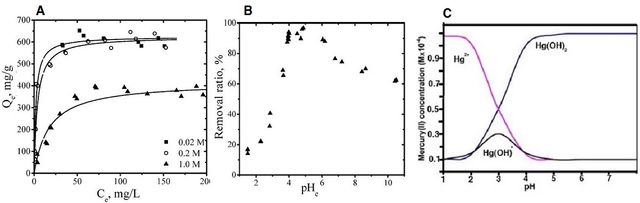
Purifying polluted water with Pb2+ and Cd2+ is a global concern, especially in the food, mining, and batteries industries. According to the World Health Organization (WHO), the maximum amount of these two heavy metals in drinkable water must be less than 0.05 mg/L and 0.005 mg/L, respectively. The exorbitant storage of lead and cadmium ions in the body harms the lungs, kidneys, skin, and blood pressure and may lead to heavy dizziness. Like hexavalent chromium and Hg2+, the pH range also affects the removal process of Pb2+ and Cd2+ [105,106]. In Figure 8A, the lead forms in the different pH were brought, and it is apparent that Pb2+ has the significant fraction in the acidic solution while Pb(OH)+ is the main fraction in the mild alkali solution and HPbO2- is the dominant fraction in the extremely alkali formation [119]. The cadmium forms could be changed by pH, as shown in Figure 8B. The Cd2+ is the principal fraction in the acidic solution, and Cd(OH)2 is the dominant form in the alkali solution [120].
On the other hand, a wide range of CPCs adsorbents was used to remove these heavy metals, particularly those with heteroatoms, especially N atoms. PANI-based adsorbents (and their derivatives) showed a remarkable removal capacity for Pb2+ and Cd2+ in pH 4–8. The dominance of H+ in severely acidic solutions and the tendency to precipitate in alkali solutions are obstacles to removing these heavy metals. Based on this observation, the chelation reaction is a probable mechanism for adsorbing, and there is a proposed mechanism in Figure 8C, in which one PANI-based composite with chitin was used as an adsorbent [120]. Therefore, augmenting the active sites of CPCs or using derivatives with more hydroxyl/amine groups might lead to better removal capacity.
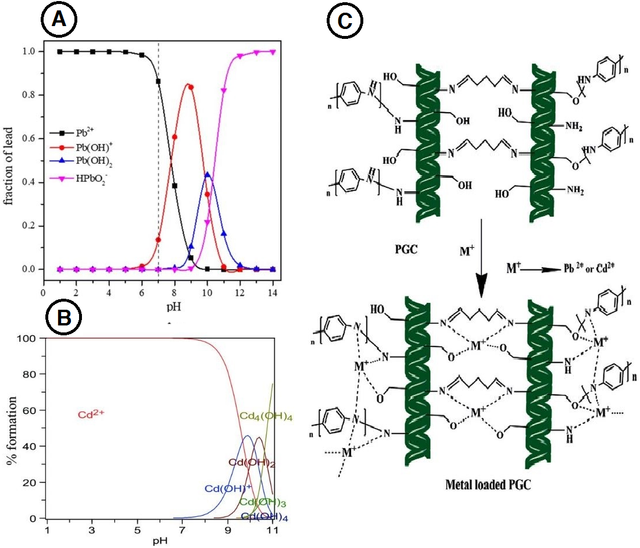
Arsenic and copper are two well-known heavy metals that have destructive effects on human bodies and the environment. Storage of copper in the body can cause severe delusion and even brain damage, and the exorbitant amount of copper is harmful to most plants. Moreover, As3+ and As5+ are toxic and have pernicious effects on the ecosystem. Unlike Hg2+, the ionic strength does not have much impact on arsenic removal, while pH and the structure of adsorbents were found to be chronic factors [105,122–124]. Many CPCs with different structures were used as adsorbents; however, PANI-based, PPy-based, and their derivatives allocated most of the research to themselves. The CPCs with metallic nanofillers like magnetic-Fe and ZnO particles showed their best removal capacity under neutral conditions.
In contrast, CPCs with PVA or MWCNT performed best in extreme alkali conditions. Hence, the complexation and chelate reaction is the most likely mechanism, and hydroxyl groups are desirable groups for polluted water with arsenic contamination [125,126]. Using PPy-based composites as an adsorbent is a powerful way to remove copper (Cu2+ and Cu4+) from aquatic solutions. Based on some reported literature, PPy-based adsorbents are more effective compared to PANI-based adsorbents regarding copper removal. Moreover, they can adsorb copper at a faster pace [125].
Although the use of nuclear industries has been limited over the past decades, there are many nuclear industries worldwide, most of which are close to water sources. Many nuclear ions are stable in the environment with a long half-life and are extremely dangerous to terrestrial and marine life. Metallic ions like Tc+4, Zr+4, U+6, and Ra+2 are the most famous radioactive atoms in aquatic systems, and removing them is chronic to protect the sustainability of human life. In addition, almost all of these metals have significant usage in other industries. Therefore, the recovery of these radioactive atoms benefits the economy [127–129]. Several CPCs were used as adsorbents to purify water with radioactive pollution, most of which are PANI-based adsorbents. Generally, the amine and imine groups of PANI and its derivatives are desirable for removing radioactive atoms. Based on previous report papers, the best removal capacity could be achieved where the adsorbents possess negative surface charges, so using zeta potential to examine the adsorbents’ surface charges is an excellent matter [130,131]. One future development in this field would be fabricating adsorbents with burnable features.
Removing dye
Technology is marching, and dyes are inseparable parts from most of them. Dyes, especially organic ones, have been used as coatings, additives, and covers, and they are essential for painting, food, machinery, and textile industries. Hence, water pollution with organic dyes is inevitable, and finding the best way to decontaminate it is vital. The question is why organic dyes are hazardous [132]. Most have at least one aromatic group in their structures, and carcinogenetic factors like nitro, amino, and azo groups are common in almost all of them [133]. Adsorbents with varied natures have been at the center of attention for years, and CPCs are among them. PANI, PPy, and their derivatives are the most common CPs regarding adsorbent de-colourization. These components are designable, open to further modification, stable in aqueous conditions, and possess many active sites. However, they are not perfect for dye purification because their surface area cannot compete with most commercial adsorbents (the surface area of PPy and PANI is around 40 and 20 m2/g). Therefore, it is essential to boost their removal capacity using PANI e and PPy as part of composites with other materials [20,26].
Various methods to fabricate CPCs with better removal capacity and techniques like grafting, blending, surface modification, and interfacial polymerization were used in research [75,80]. Hence, sorting these CPCs is challenging, but grouping them into organic and inorganic types is common. The CPCs with mineral clays, metals, metallic carbides, metal-organic frameworks, zeolite imidazole frameworks (ZIFs), and metal oxides (like ZnO and Fe3O4) in their structures are in the inorganic-type composites. In addition, CPCs with carbon-based hybrid materials (like graphene oxide (GO), multi-wall carbon nanotubes (MWCNT), graphitic carbon nitride (C3N4)), biopolymers, bio-waste, and other polymers in their structures are in the organic/hybrid-type composites [59]. As shown in Figure 9, the SEM and TEM images of different CPCs can be observed, indicating that the final morphology varies from type to type.
Inorganic-type CPC
There are a few reports about incorporating zero-valent charge (metal nanoparticles) into CPs for water remediation. Most similarly fabricated composites with this approach were used in the subjects where enhancing conductivity was the main point. However, incorporating metal oxides, especially magnetic metal oxides, into PANI or PPy is a well-established approach to water purification. Generally, embedding magnetic nanoparticles can enhance surface area and removal efficiency. In addition, facile operation, low cost, and effective separation are the merits of CPCs with magnetic components. Core-shell composites catch so much attention because their magnetic core can respond to outer stimuli. At the same time, polymeric shells can contain functional groups and increase compatibility with organic matrices [143,144]. Both copolymerization and interfacial polymerization (IP) were used to fabricate magnetic CPCs. Among CPs, PANI and its derivatives were used remarkably to fabricate magnetic CPCs [145]. As shown in Figure 10, porous dandelion-like NCs can be prepared via PANI and two metal oxides, including magnetic ones.
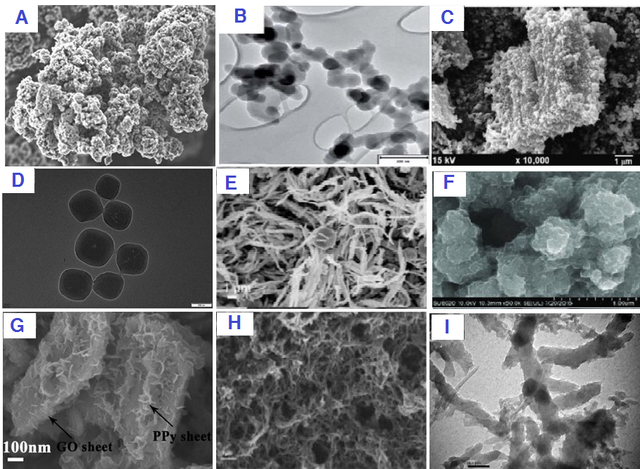
Moreover, it was found that PANI-derivatives with reach OH, NH2, and COOH functional groups (in both backbone and pendent groups) have more removal capacity compared to PANI itself. This observation reveals that the removal mechanism is probably governed by redox reaction or complexation [81,82]. Eventually, it can be said that magnetic CPCs are better adsorbents than bare CPs, based on evidence [146]. Furthermore, the dye removal efficiency of magnetic PANI-based adsorbents was investigated, and the results indicated that the purification process is an exothermic reaction. Hence, increasing the solution temperature can dwindle the magnetic CPC’s removal capacity, and r.t is the best for purifying the water from the dye [147].
Another remarkable inorganic-type CPCs is silica-based. A wide range of silica-based CPCs was used in water treatment because they are affordable, have a high surface ratio, can enhance the total porosity, and possess many hydroxyl groups. These features can help silica-based CPCs react with most dyes and trap heavy metals and organic pollutants like poly-fluoroalkyl substances (PFAS) and perfluorooctane sulfonic acid (PFOS). The high porosity of silica-based composites can increase the lifetime and reusability of adsorbents, which is a beneficial attribute in reducing the capital cost of adsorbents. Another feature that makes silica a perfect candidate for compositing is that it can offer too many options, and researchers can opt based on their needs. For example, aluminosilicate (zeolite), ZIF, silica gel, mesoporous silica, and clinoptilolite are some of the examples, but not all of them [148,149].
Clay minerals are one of the largest inorganic groups in the water and waste treatment field. Clay minerals are affordable and abundant, and they can boost porosity. Fabricating composites based on PANI and PPy with clay minerals has been reported for decades. For example, chlorite, bentonite, illite, smectite, and montmorillonite are well-known mineral clays. Generally, most mineral clay-based CPCs were used for dye removal rather than heavy metal or salt purification, maybe because the pores of these composites are relatively wider than silica-based CPCs [150,151].
MXenes are among the most recent materials that are gaining more and more attention in all fields, including water treatment. These two-dimensional nanosheets have accordion structures and negative surface charges. They have many types, and having the option to choose the right one based on the need is a privilege. MXene-based adsorbents were used to purify pollutants like dyes, heavy metals, salts, and PFAS because they have a significant surface area, numerous functional groups, including hydroxyls, and available active sites. However, they are expensive and have poor stability in the aqueous solution, so compositing them is a common approach. Other papers in which PPy and PANI have reported MXene-based CPCs and their derivatives are the most common. Preparing the condition where PANI and PPy possess positive surface charges is a reliable approach for fabricating MXene-based composites. This criterion also benefits keeping the order of MXene layers. Mxene-based CPCs are desirable for dye removal because the redox reaction of Ti+4 to Ti+3 is a powerful tool for degrading organic-type molecules, especially azo ones [152,153].
Organic/hybrid-type CPCs
A favorite combination is using reduced graphene oxide (r-GO), GO, activated carbon (AC), and MWCNT with other materials to fabricate composites. These porous carbon-based materials have excellent thermal stability, high surface area, and available functional groups, and their two-dimensional structure is a favorite for further modification. PPy, PANI, and their derivatives are CPs used with these carbon-based materials to decontaminate the water with different pollutants. Based on the published paper, a heterogeneous adsorptive pattern is most likely for the PPy@AC composite, and removal capacity was boosted by nearly 90% after coating AC on the PPy. Another reported paper revealed that GO alongside PANI means higher dispersing, which is a favorite because CPs are insoluble. Dispersion is the critical factor in fabricating stable composites. In addition, analyses revealed that PANI@GO nanosheets might adsorb different dyes via π-π interaction, van der Waals, and dipole-dipole interaction [154–156].
Waste to use is a riveting term in modern life, and countless research groups worldwide are working on this subject to benefit their communities. Waste, specifically agricultural waste, is cost-effective, abundant, and biocompatible, so using biomass in water treatment is one of the hottest subjects in water remediation. Rice husk, Sawdust, Alstonia scholaris leaves (AL), Basil seed, and many others were used to fabricate conductive polymer-based composites. The removal efficiency of AL@PANI composites was examined, and the data revealed that composites could purify diamond green (a kind of dye) from water 10 times better than PANI. The proposed adsorption mechanism for this fascinating composite is hydrogen bonds, π-π interaction, and dipole-dipole interaction. Sawdust (SD) is a by-product in the wood industry, and its composition with PANI was used to purify the aqueous system from heavy metals, dyes, and even oils. Surprisingly, the BET test confirmed that using SD could augment the specific surface area of PANI by more than 11 times. The mild acidic solution and r.t are the best conditions to boost the removal capacity of PANI@SD composites [157,158].
Biopolymers like cellulose, chitosan, starch, and other polysaccharides reel more eyes into themselves. They are cheap and, most importantly, green, and in a world where green chemistry is catching more attention, using biopolymers to fabricate composites is chronic. Most polysaccharides have abundant functional groups, including hydroxyl ones, which are excellent for complexation, chelation, and redox reactions. However, due to the large size of these biopolymers, they easily interact with other particles. Therefore, synthesizing biopolymers on a nanoscale is a serious concern. Preparing biopolymer-based CPCs was a common and sought-after subject in previous decades, where PANI and its derivatives had the most fraction [159,160].
Dye removal mechanism
Like heavy metal removal, dye removal with CPCs could also be affected by numerous factors, including functional groups of dye and CPCs, adsorbent dosage, solution temperature, solution pH, and the structure of the dye. The type of interaction between the adsorbents’ surface and the dyes’ surface determines the final mechanism. However, hydrogen bonds, dipole-dipole interaction (van der Waals forces), and π-π stacking interaction control the overall mechanism [161,162].
On the other hand, most industrial dyes have negative surface charges; the most well-known anionic dyes are sunset yellow, methyl orange, and Congo red. Therefore, CPCs, particularly PANI-based ones in an acidic solution, could boost removal efficiency. As mentioned earlier in other sections, PANI has positive surface charges in the pH range below five and sees its peak around four because, in this range, its amine groups can be pronated and turned into –NH3+. The exact mechanism and explanation are also accurate for PPy). In addition, CPCs adsorbents like PPy, PANI, PTh, and their derivatives can adsorb cationic dyes with π-π stacking interaction or hydrogen bonds [163,164].
Pharmaceutical removal
Removing by-products of pharmaceutical industries like drugs and eliminating personal care from aqueous systems is a serious concern, especially in crowded cities. Residual antibiotics in the water can damage the environment’s sustainability and human bodies. Quinolone is a large group of antibiotics used globally at a staggering speed. Most of the quinolone is fluoroquinolone, so removing these fluoro-based antibiotics is necessary. Most drugs’ molecular weight, size, surface charges, and structures, including fluoroquinolone, are similar to dyes, so their removal is quite similar. In addition, almost all pharmaceutic by-products have an organic nature, another crucial similarity to organic dyes [165,166]. Hence, several research groups used PANI-based or PPy-based CPCs to purify water from these pollutants. Based on reported papers, the main mechanisms are π-π stacking interaction or hydrogen bonds. Furthermore, sulfonamide groups in drugs and antibiotics are common, and this group can also be expunged from water sources with the exact mechanism and CPCs [167,168].
Other common pollutants
Human activity and creating pollution are a perfect completion. Besides, water resources are vital to most industries and activities, so water pollution is inevitable in most cases. In addition to heavy metals, salts, pharmaceutical by-products, and dyes, other important pollutants include phenolic compounds, perfluoroalkyl, PFAS, and PFOS [169–171].
Releasing phenolic compounds to water supplies is possible in many industries like food, petrochemicals, and mining. Phenolic groups are among the most toxic compounds. Removing them from water is a top priority based on the Environment Protection Agency (EPA) because they have at least one aromatic group in their structures, and phenolic groups are super active. These compounds can harm the lungs, cause high obesity, and interrupt the enzyme’s function. Resorcinol and p-nitrophenol are among the most common phenolic compounds, and expunging them via CPCs was reported. PANI-based adsorbents, especially those with metal oxides, were used to purify water from phenolic groups, and there is a report that phenolic adsorption is an endothermic reaction [172,173].
PFAS/PFOS are gaining much attention, and several approaches have been developed to remove these new generations of pollutants. The problem is that they are everywhere. They are part of cleaning products, dental floss, shampoos, coatings, waterproof fabrics, grease-resistant papers, and others. Although ion exchange resins have been used widely to remove these particles from water supplies, nowadays, many composites have found their way in this field. CPCs with porous carbon-based materials are among these composites. PFAS/PFOS removal is still a new subject, but based on the published papers, van der Waals forces and π-π stacking interaction are the most probable mechanisms [174].
CPCs for photodegradation
Using UV or visible light to eliminate pollution from water sources is an affordable, facile, and green approach. CPs were used alongside other materials like semiconductors for a possible synergistic effect on water remediation. It was found that CPs could endow merits to composites with photodegradation ability due to their organic nature, processability, and conductivity. Therefore, increasing the life span of composite, preventing photo corrosion, and boosting the decontamination effect are what CPCs can contribute to composites. PANI, PPy, PTh, and their derivatives are common CPs to fabricate composites for photodegradation [175–177].
Photo-generated electron-hole pairs are chronic for photodegradation. Enhancing their recombination is key. Using PANI and its derivatives can boost this recombination and broaden the light adsorption window. This unique feature makes PANI (and its derivatives) a perfect polymer for this field of work. PANI was blended with a wide range of metal oxides and mineral clays during the past years to fabricate favorite composites. PANI/TiO2 or PANI/ZrO2 are among the most common combinations in this area. Using PANI can solve the agglomeration of TiO2/ZrO2, boost photocatalyst reaction, and enhance the removal capacity of both heavy metals and dyes. PPy is the same as PANI and has been blended with various metal oxides. In addition, there is a report that radiolysis is the best way to boost PPy-based composites for photodegradation [178–180].
CPC as membranes
Membranes are among the leading choices in water and wastewater treatment because they can eliminate almost all pollutants, from salts and heavy metals to dyes and PFAS. Membranes have two mechanisms for removing water contamination: a sieving mechanism and an adsorption/desorption mechanism. Antifouling and antibacterial surfaces are vital to increasing membranes’ efficiency or life span. In addition, polymeric membranes caught most of the market because of their easy fabrication, abundant choices, cost-effectiveness, easy surface modification, and many other attributes. The best membranes are those with selective recognition of stimuli, mainly called smart membranes. These membranes can trigger responses to external stimuli like pH, ionic strength, vibration, magnetic forces, temperature, pressure, and oxidation/reduction reactions [181,182].
CPCs, particularly PTh, PPy, PANI, and their derivatives were widely used in the membrane for various applications, from batteries and sensors to bioengineering and water treatment. Using these polymers can lead to the fabrication of smart membranes with perfect antifouling features and electro-response membranes [183,184]. CPCs were used to fabricate various membranes for filtration purposes, including microfiltration, ultrafiltration, nanofiltration, forward osmosis, and reverse osmosis. The CPs can control the pore size of the membrane surface, which is a principal feature of both water permeation and rejection rates. Moreover, by applying an electrical field around the membrane, there is a good chance for self-cleaning the surface, which can expand the membrane’s life and efficiency in long-term applications. Grafting, blending, or polymerization of PPy and PANI with or into polymers like polyethersulfone, polysulfone, polyether block amide, and polyvinylidene fluoride are the approaches that CPs have been used [185–187].
Obstacle of using CPCs in water treatment
Although CPCs are fabulous materials in many fields, especially those that require electrical conductivity, their usage in water treatment still needs to overcome many challenges. One of the main problems is that the exact relationship between morphology, removal mechanism, mass ratio, and other factors needs more specific and much more research. Low-solubility and the fact that dispersion is the only reliable way to fabricate most composites is one of the most significant challenges. On the other hand, melting these polymers is a massive problem because almost all of them would be degraded before melting point, so using this technique for compositing is often off the table, and many composites require high temperatures. CPCs are considered cheap compared to most materials. However, compared to other polymers, they are not that affordable, and competing with other polymer-based composites for water treatment is not economical.
Even though some PANI and PPy derivatives were developed with higher surface area and more available functional groups, CPs cannot surpass most commercial polymers in the water treatment fields. They might not be the first choice to use in related composites. Furthermore, using these composites where there is high pressure is limited. Because most CPs, especially PTh and PPy, do not have excellent mechanical stability, even though compositing them is a feasible technique to reduce the impact of this defect, they cannot compete with other similar polymers.
Conclusion
An in-depth study of conductive polymer composites (CPCs) is presented here, elucidating their distinctive characteristics and classifications. CPCs have substantial advantages when applied to energy storage, photovoltaic devices, and semiconductor technologies. Despite their potential utility in water treatment processes, their capabilities and mechanisms of action remain relatively underexplored. Composing CPCs with various materials has made significant advances in recent decades, including porous carbon, metals, metal oxides, metal carbides, mineral clays, bio-waste, biopolymers, and synthetic polymers. By developing these composites, CPCs can enhance their functional properties and performance. As a result of the incorporation of such materials, the surface area, the availability of functional groups, the porosity, the thermal stability, and the mechanical robustness of the materials have all been increased, all of which contribute to the increase of contaminant removal efficiency. Different types of CPCs are used for environmental remediation, but adsorbents are the most extensively studied. They are gaining prominence because of their straightforward design and versatility in application. Several critical factors influence adsorbent effectiveness, including pH levels, adsorbent doses, ion strength, solution temperatures, and initial contaminant concentrations. Understanding how these variables interact to optimize adsorption processes and maximize contaminant removal is essential. The demand for innovative filtration technologies incorporating self-cleaning functionality and enhanced antifouling properties has led to the development CPCs membranes and adsorbents. Several studies have demonstrated the effectiveness of CPCs membranes in removing a wide range of pollutants. In addition to heavy metals such as hexavalent chromium and Hg2+, organic dyes, PFAS, pharmaceutical residues, and phenolic compounds, there are also a variety of other contaminants to consider. The predominant mechanisms underlying heavy metal removal typically involve complexation and redox reactions. At the same time, the elimination of organic dyes and pharmaceutical contaminants is likely facilitated by van der Waals forces and π-π stacking interactions. Although CPCs can be used in many ways to treat water, substantial research is needed to utilize their capabilities better and fully exploit their potential in this critical area of environmental remediation. In the future, studies should examine the mechanisms of interaction between CPCs and various contaminants and explore novel composite materials that may enhance the effectiveness of these polymers.
Statements and Declarations
Competing Interests
The author declares no competing interests.
Ethics approval
Not applicable.
Data availability
Not applicable.
Funding
No funding was received to support this work.
Acknowledgments
Support for this investigation by the Iran University of Science and Technology is gratefully acknowledged.
Author Information
Mohammad Mehdi Salehi—Catalysts and Organic Synthesis Research Laboratory, Department of Chemistry, Iran University of Science and Technology, Tehran 16846–13114, Iran.