Visual Abstract
Abstract
This research aimed to investigate the role of different isomers of transforming growth factor-β (TGF-β) in the up/downregulation of chondrocyte markers. The monolayer culture of the chondrocyte resulted in the dedifferentiation of cells and the production of stress fibers, which was prevented by high-density and 3D chondrocyte culture. This work demonstrated a successful and typical cell engineering of chondrocyte monolayers with our model using isolated primary rat articular chondrocytes (RAC) through cell seeding, 2D cell expansion, and differentiation in vitro, without the use of a bioreactor. Therefore, the same model can be used for the isolation of human articular chondrocytes (HAC) from clinically approved human biopsies for autologous chondrocyte implantation and/or monolayer production (e.g., cytotoxicity tests). Our model can also be used for the direct expansion of the limited number of chondrocytes obtained from RAC or HAC, reaching higher densities similar to those used in conventional cartilage tissue engineering based on cells expanded by 2D cultures. Our results indicated that the combination of transforming growth factor-beta (TGF-β), including TGF-β1, 2, and 3, increased the production of Collagen Type-II. Additionally, manipulated TGF-β1, 2, and 3 negatively regulated cell proliferation. The amount of chondroitin sulfate proteoglycan (CSPG) and laminin were reduced by all three isomers of TGF-β and their manipulated forms, but the expression of fibronectin and S-100 proteins was not significantly affected by TGF-β isomers. Our results suggest that TGF-β1 and 2 could be utilized in the fabrication of biodegradable scaffolds for 3D chondrocyte culture due to their ability to induce cell proliferation
Introduction
Cartilage is an avascular tissue [1,2] where oxygen and nutrients are delivered through the synovial fluid [3,4]. This contributes to the poor repair characteristic of cartilage [5,6], as even small lesions can cause severe mobility problems for patients [7,8]. To understand how cartilage injuries can be treated in vivo [9], it is important to examine the behavior of chondrocytes about model wounds [10] and the presence of various cell stimulators [11], such as different growth factors, in vitro [12]. Cytokines are highly potent biomolecules that regulate cellular functions and play multiple roles in disease initiation and inhibition [13]. The transforming growth factor-β (TGF-β) superfamily, a group of pleiotropic cytokines, has a high molecular weight of 25kD and consists of homodimeric peptides [14,15]. These specialized macromolecules actively control cellular processes including proliferation, apoptosis, cell migration, and adhesion [16]. TGF-β1, 2, 3, 4, and 5 participate in signal transduction between the extracellular environment and the nucleus [17,18], with three of these cytokines being particularly influential in cellular development [19,20].
The limited repair capacity of cartilage is directly associated with chondrocyte cells, their adhesion, proliferation, and migration into the wound site [21]. Some growth factors, like TGF-β1, are involved in scarless repair of mature cutaneous tissue [22,23]. However, there have been few evaluations of the effect of transforming growth factor-βs and their manipulated forms on chondrocyte cell proliferation and cartilage wound repair. Transforming growth factor-betas are produced in an inactive/latent form by almost all cell types [24,25], suggesting a regulatory role in most tissues. While the function of TGF-βs has been investigated in various cell types, their role in cartilage repair remains not fully understood. Many studies on transforming growth factor's effect on chondrocytes are not specifically related to cartilage wound repair. The TGF-β superfamily consists of various types of polypeptides that regulate the upregulation and downregulation of membrane proteins, influencing cell adhesion, proliferation, differentiation, activation, migration, and apoptosis. Stimulation by TGF-β1 has been reported to increase the synthesis of cartilaginous matrix and play an important role in cartilage repair by promoting cell proliferation rates, migration, and adhesion [11,19]. This indicates how cartilage repair is initiated and how it can be accelerated by transforming growth factor-beta1 [10]. Chondrocytes can be induced to obtain a chondrocyte morphology by seeding at high cell density [26], and a fibroblastic morphology by culturing at low density after isolation.
We understand that the addition of TGF-β1 in particular, leads to the downregulation of cell proliferation, adhesion, and migration, which are essential for wound repair. Based on our previous findings, we believe that a regenerative approach utilizing cartilage cell therapy in combination with growth factors (such as TGF-β1&2) as stimulants could promote cartilage repair [27–29]. We also believe that wound healing of articular cartilage due to injury or disease could be achieved by providing a suitable matrix that supports the repair process. Tissue-engineered cartilage, using isolated chondrocytes and biomimetic or bio-responsive hydrogels with a precise selection of cytokines (e.g., TGF-β) [30,31], is one such approach. Scaffolds were used to deliver cells and promote the formation of the cartilage matrix, employing biodegradable materials. Therefore, the goal of this research is to investigate the role of different isomers of transforming growth factor-β (TGF-β) in the upregulation and downregulation of chondrocyte markers.
Materials and methods
TGF-β preparation
Transforming growth factor-β is a multifunctional peptide that is soluble in an acid solvent. While it is also soluble in water, the solution would be sticky and difficult to aliquot and utilize. TGF-β requires a carrier molecule, such as BSA, to enable cell uptake. For this study, the human recombinant transforming growth factor (Sigma Aldrich, UK) was diluted according to supplier recommendations using hydrochloric acid (HCl) and bovine serum albumin (BSA). According to the supplier instructions, 10mg of bovine serum albumin was dissolved in 10 ml of 4 mM hydrochloric acid to obtain a concentration of 1mg/ml HCl/BSA. The solution was then sterilized using a 0.22µm filter. Individual 2μg aliquots of TGF-β1, 2, and 3 were dissolved in 400 µl of 1mg/ml HCl/BSA, divided into 40 10μl vials, and stored at -20ºC.
Cell culture
Primary chondrocyte cells, isolated from the knee joint of a neonate Sprague-Dawley rat, were cultivated using a planar culture system. The cells were expanded 200-fold through four passages and used for this experiment. Initially, cartilage was extracted from the articular joint of a neonate Sprague-Dawley rat, and the cartilage tissue covering the epiphysis at the knee joint was pre-digested in 4ml of trypsin, followed by further digestion in 1ml of type-1 collagenase. The isolated chondrocytes were then centrifuged, and the pellet was re-suspended in 15ml of fresh media and cultured in a monolayer in a 75cm² tissue-culture flask until achieving 70-80% confluency. The cells were quantitatively expanded in a monolayer, and after the third passage, the chondrocytes were trypsinized, poured into 15ml centrifuge tubes, and centrifuged at 2000rpm. The supernatant was discarded, and 5ml of fresh media was added to the pellet, which was then re-suspended and cultured in a 25cm² tissue-culture flask.
A sterilized 22cm² glass coverslip was placed into a sterile Petri dish. A total of 300µl of chondrocyte cell suspension, with a cell density of 20x104 cells/ml and supplemented with 10ng/ml TGF-β1, was seeded on the glass coverslip. The coverslip was sealed with parafilm and incubated at 37°C for 24 hours. The same amount of cell suspension, with the addition of TGF-β2, TGF-β3, and manipulated forms of TGF-β (TGF-β1&2, TGF-β1&3, TGF-β2&3, and TGF-β1&2&3), was prepared and seeded on separate glass coverslips, then incubated at 37°C. A positive control culture without the addition of any type of TGF-β was also prepared using 300µl of cell suspension on a glass coverslip, followed by incubation at 37°C. For each treatment, a separate positive control culture was prepared to monitor and compare the results.
The reagents used during the isolation and purification process included cell culture medium (DMEM), Hank's Balanced Salt Solution (HBSS), Trypsin, and Collagenase (all purchased from Sigma, UK). Dulbecco's modified eagle medium (DMEM), a sterile-filtered cell culture medium, was used and supplemented with 20% v/v fetal calf serum (FCS), 2.5 U/ml L-glutamine, 100 U/ml penicillin, 0.1 mg/ml streptomycin, and 1μg amphotericin B (Fungizone) (Sigma Aldrich, UK). Additionally, 70% ethanol was prepared and used for sterilizing tools, tubes, and flasks. Passage 4 was used for this experimental work.
Antibodies used in this research work
The antibodies used in this research include Anti Chondroitin Sulfate Proteoglycan-5 (CSPG-5) (Sigma-Aldrich, UK), Monoclonal anti-S-100 (Sigma Aldrich, UK), Monoclonal anti-fibronectin antibodies (Sigma Aldrich, UK), Monoclonal anti-Collagen Type-II A1 (mouse IgG1) (Sigma Aldrich, UK), and a peroxidase detection kit for laminin (Sigma Aldrich, UK).
Monoclonal anti-S-100 (β-subunit, mouse IgG1 isotype) (Sigma Aldrich, UK) was used to detect the S-100 marker. The as-received S-100 antibody had to be thawed, aliquoted in 10 µL, and immediately stored back at -20ºC, as specified by the supplier. A total of 50 mg BSA was dissolved in 5 ml PBS or HBSS to produce a 1% BSA w/v solution. 10 µL of the primary antibody was then added to 4,990 µL of PBS or HBSS to make a 5ml primary antibody solution with 1% BSA. Only 250-300 µL was required for each coverslip, so 5 ml was used to stain up to 20 coverslips.
The standard protocol of immunocytochemical staining was followed for the detection of S-100 and collagen type-II biomarkers. Before immunostaining, the permeability of the cell membrane was increased by using 0.1% Triton X-100, a non-ionic surfactant used for solubilizing proteins (Sigma Aldrich, UK), followed by the standard protocol described below:
The anti-S-100 primary antibody and anti-collagen Type-II antibody with a dilution ratio of 1:500 were prepared separately. 20 μL of the primary antibody was added to 10 ml of 1% HBSS. The coverslips were carefully washed three times for five minutes each with 6 ml of HBSS on a shaking platform at room temperature. The third HBSS wash was left for 30 minutes in the Petri dishes. After aspirating the HBSS, the area around the coverslips in the Petri dishes was dried carefully. The secondary antibody was prepared by using a common ratio of 1:400. According to the supplier's instructions, the final concentration of the secondary antibody needed was 1-10 μg/ml, while the delivered antibody had a concentration of 2mg/ml.
The Petri dishes were incubated with the secondary antibody for two hours at room temperature in a dark and humid environment. After the two-hour incubation, the Petri dishes were washed three times for 15 minutes each with 6ml of HBSS on a shaking platform at room temperature. The Petri dishes were mounted, leaving the last HBSS in them, and incubated at four degrees in a dark and humid environment.
Peroxidase staining for Laminin
Laminin from the basement membrane of Engelbreth-Holm-Swarm mouse sarcoma (Sigma Aldrich, UK) was diluted with HBSS at a ratio of 1:10000 and stored at -20°C. Before staining the laminin, the primary chondrocytes cultured on 22cm² glass coverslips were fixed using a 1% formaldehyde solution. The coverslips were then treated with two drops of rabbit anti-laminin antibody (primary antibody) for one hour. Afterward, the coverslips were washed three times with PBS. The PBS was completely aspirated, and two drops of biotinylated secondary anti-laminin antibody (Sigma Aldrich, UK) were added. The coverslips were washed three times for 15 minutes each with PBS, treated with peroxidase, and incubated at room temperature for 20 minutes.
According to the supplier's instructions, a mixture of solutions was prepared, consisting of 4ml deionized water, two drops of acetate buffer, one drop of AEC chromogen, and one drop of 3% hydrogen peroxide (Sigma Aldrich, UK). After the 20-minute incubation, the coverslips were washed once with PBS, and two drops of the prepared solution were added to the coverslips and incubated for 10 minutes. The staining process was monitored under a light microscope during the 10-minute incubation. After incubation, the samples were washed with PBS, imaged, and saved for future analysis.
The experiment was performed under sterile conditions in a vertical laminar hood, and it was repeated three times to ensure the precision of the results. The level of stained cells was compared to the control to evaluate the effect of various forms of TGF-βs on the up-or down-regulation of stained proteins. After 24 hours, each chondrocyte culture was fixed with a 1% formaldehyde solution and immunocytochemically stained for Collagen Type-II, chondroitin sulfate proteoglycan (CSPG), S-100 protein, and fibronectin. Laminin, which is a membrane protein regulating cell adhesion and migration [32], was stained using the peroxidase staining kit for laminin (Sigma Aldrich, UK) to determine its localization.
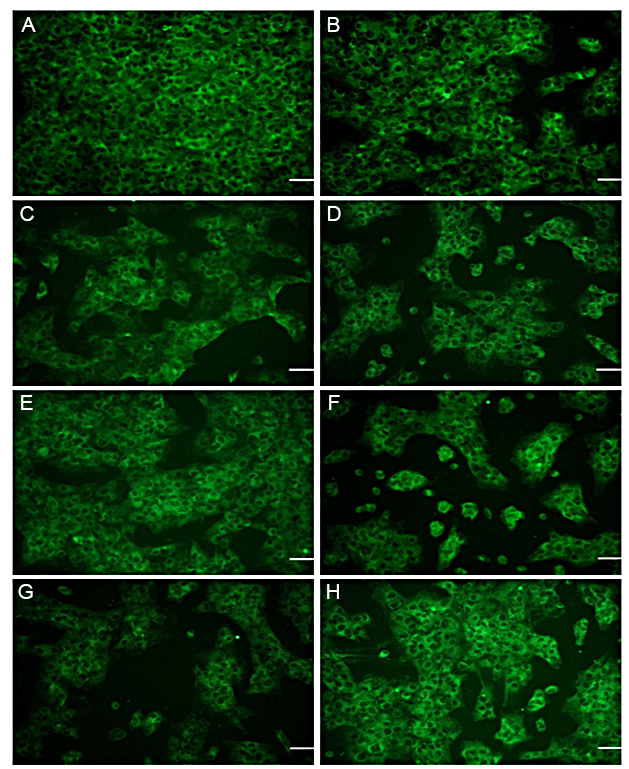
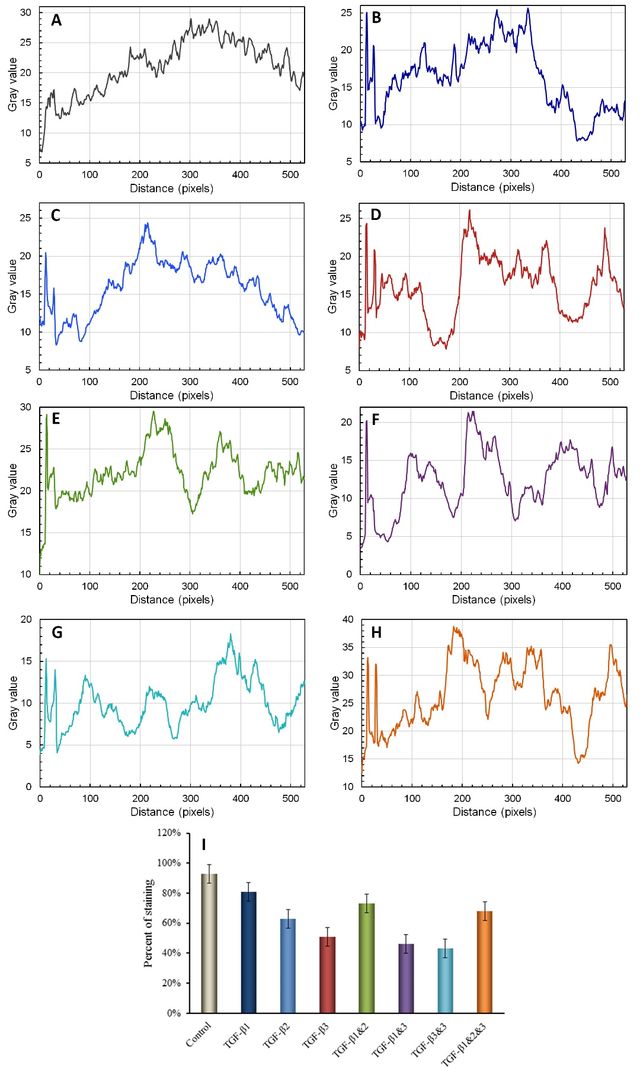
Statistical and quantitative analysis
The mean percentage of stained cells per treatment group was tested for normality using a Kolmogorov-Smirnov test. For normally distributed data (p > 0.05), analysis was performed using SPSS through a One-Way Analysis of Variance (ANOVA), followed by a post-hoc Bonferroni test. Non-normally distributed results (p < 0.05) were analyzed using the Kruskal-Wallis test and serial Mann-Whitney tests. A P-value of <0.05 was considered indicative of a significant difference in the statistical analysis. The P-value obtained from pairwise comparisons of the quantitative graph of immunofluorescence staining of high-density chondrocytes for Collagen Type II, CSPG, and S100 in cultures treated with TGF-β1, TGF-β2, TGF-β3, TGF-β(1+2), TGF-β(1+3), TGF-β(2+3), TGF-β(1+2+3), control, and all possible combinations was analyzed accordingly. An asterisk (*) denotes pairs that are significantly different from each other, with * indicating a P-value < 0.05, ** indicating a P-value < 0.01, and *** indicating a P-value < 0.001. The Java-based image processing program ImageJ developed at the National Institutes of Health and the Laboratory for Optical and Computational Instrumentation, was used to generate the quantitative analysis of immunostaining images and plot the results.
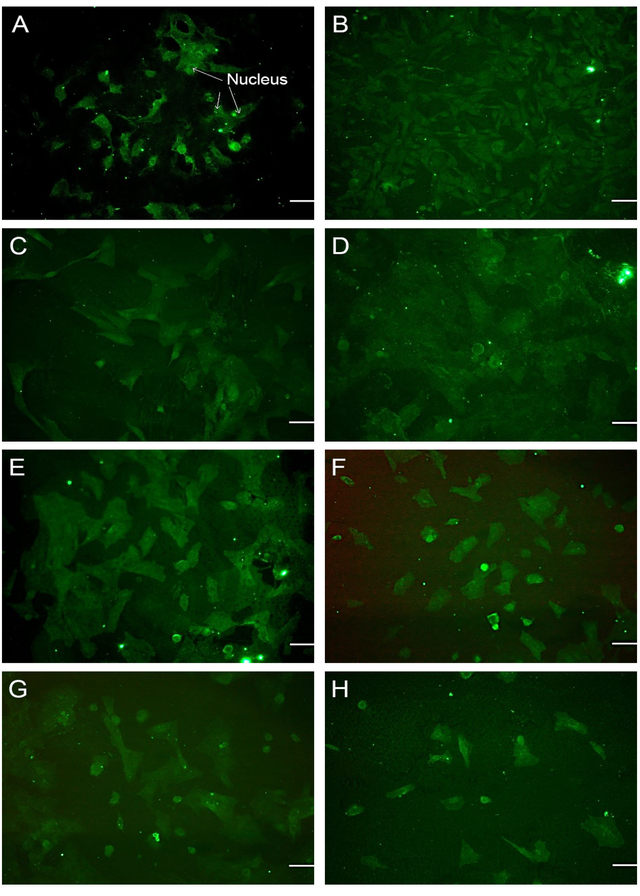
Results and Discussion
Collagen Type-II
The up/downregulation of Collagen Type-II production, a predominant protein involved in cartilage formation [33], was analyzed in the presence of transforming growth factor-β (TGF-β). TGF-β works in conjunction with extracellular matrix (ECM) molecules to regulate chondrogenic differentiation and the development of cartilage [25]. The effect of TGF-β and its manipulated forms on the synthesis of Collagen Type-II, a marker for chondrogenesis [34], was examined. The results indicated strong staining for Collagen Type-II in primary chondrocytes cultured in media supplemented with a mixture of TGF-β1, TGF-β2, and TGF-β3, as depicted in Figure 1-A and H. A significant difference was observed between the control group and the groups treated with TGF-β1, TGF-β2, or TGF-β3 (P > 0.001). Manipulation of TGF-β2 and TGF-β3 resulted in a reduction in Collagen Type-II staining (see Figure 1A-G, Figure 2).
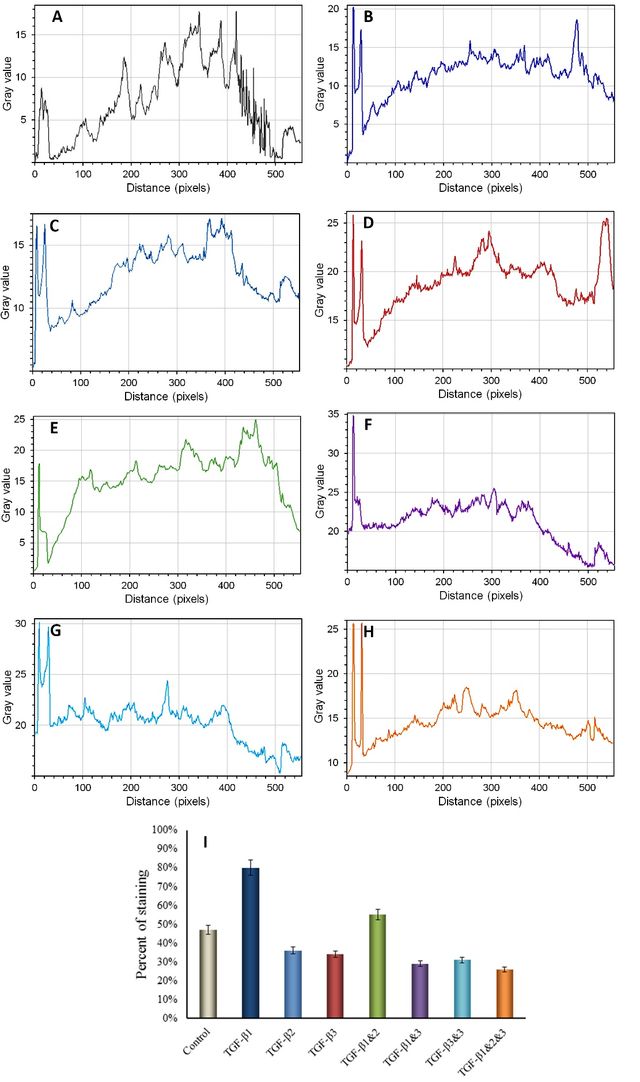
Chondroitin Sulfate Proteoglycan (CSPG)
The results obtained from the immunofluorescence staining of chondroitin sulfate proteoglycan (CSPG) revealed that CSPG staining was dispersed on the extracellular matrix (ECM) of chondrocytes when cultured in media supplemented with manipulated forms of TGF-β2, TGF-β3, TGF-β1&2, and TGF-β2&3 (see Figure 3-C, D, E, and G). Strong staining of CSPG was observed in media without any addition of TGF-β, as depicted in Figure 3-A. However, the mediums containing TGF-β1 and TGF-β2 exhibited lower levels of CSPG staining (See Figure 3-B, C and Figure 4). Immunofluorescence staining of CSPG demonstrated that this protein was localized in the nucleus of chondrocytes in all cases, as shown in Figure 3.
S–100
The evaluation of the effect of different types of TGF-βs and their manipulated forms on the synthesis of S-100, a chondrocyte marker, revealed that this protein was distributed along the extracellular matrix (ECM). There was no significant impact on the up/downregulation of CSPG, except for slightly stronger staining observed around the nucleus and cell membrane, as demonstrated in Figures 5 and 6.
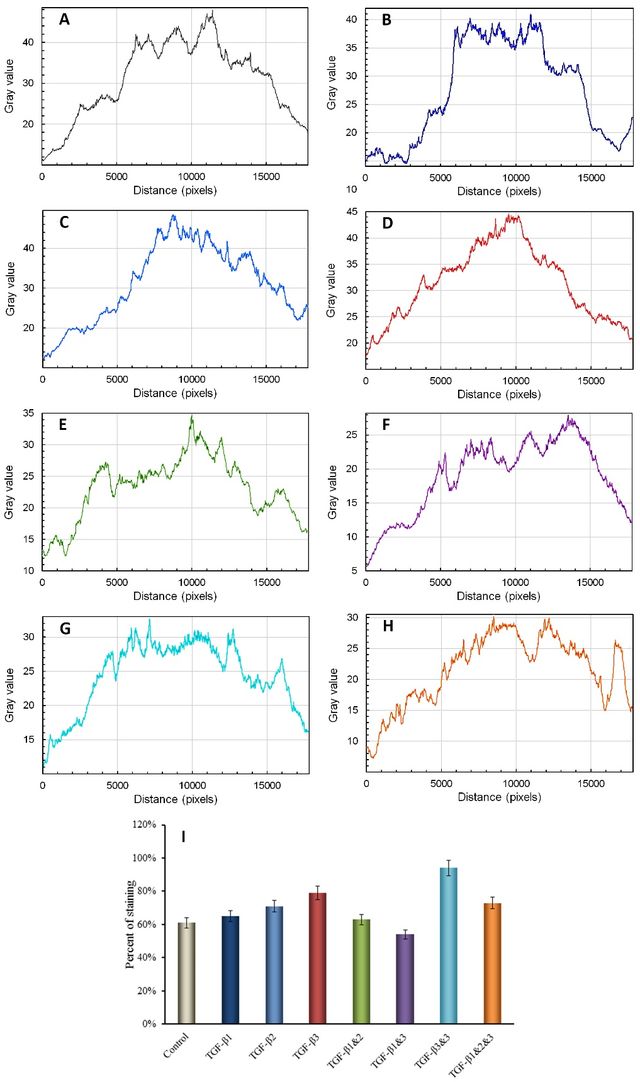
The combination of TGF-β1&2&3 increased the production of Collagen Type-II. Additionally, manipulated TGF-β1&2&3 negatively regulated cell proliferation. The levels of chondroitin sulfate proteoglycan (CSPG) and laminin were reduced by all three types of Transforming Growth Factor-β (TGF-βs), as well as their manipulated forms. However, the expression of fibronectin and S-100 proteins were not significantly affected by TGF-β isomers (refer to Tables 1 and 2).
The results obtained from immunocytochemical staining for the localization and expression of laminin indicated that TGF-β and any manipulated form of this growth factor slightly reduced laminin expression in the chondrocyte monolayer. The highest level of staining was observed in the control culture, while the lowest expression was seen in chondrocytes cultured in TGF-β1&2, TGF-β1&3, and TGF-β1&2&3-supplemented media. Chondrocytes cultured in media treated with TGF-β2&3 exhibited a fibroblast-like morphology and slightly more staining for laminin. Laminin is primarily localized and spread within the extracellular matrix of the cells.
Treatments | Collagen Type-II | CSPG | S100 |
Control | 93% | 47% | 61% |
TGF-β1 | 81% | 80% | 65% |
TGF-β2 | 63% | 36% | 71% |
TGF-β3 | 51% | 34% | 79% |
TGF-β1&2 | 73% | 55% | 63% |
TGF-β1&3 | 46% | 29% | 54% |
TGF-β2&3 | 43% | 31% | 94% |
TGF-β1&2&3 | 68% | 26% | 73% |
Treatments | Collagen Type-II | CSPG | S100 | |||
P-value | Sig diff | P-value | Sig diff | P-value | Sig diff | |
Control—TGFβ1 | 0.001 | *** | 0.001 | *** | 0.338 | |
Control—TGFβ2 | 0.001 | *** | 0.001 | *** | 0.001 | *** |
Control—TGFβ3 | 0.001 | *** | 0.001 | *** | 0.001 | *** |
Control—TGFβ1+2 | 0.002 | ** | 0.025 | * | 0.338 | |
Control—TGFβ1+3 | 0.001 | *** | 0.001 | *** | 0.005 | * |
Control—TGFβ2+3 | 0.001 | *** | 0.001 | *** | 0.001 | *** |
Control—TGFβ1+2+3 | 0.001 | *** | 0.001 | *** | 0.001 | *** |
TGFβ1 - TGFβ2 | 0.001 | *** | 0.001 | *** | 0.005 | * |
TGFβ1 - TGFβ3 | 0.001 | *** | 0.001 | *** | 0.001 | *** |
TGFβ1 - TGFβ1+2 | 0.001 | *** | 0.001 | *** | 0.148 | |
TGFβ1 - TGFβ1+3 | 0.001 | *** | 0.001 | *** | 0.001 | *** |
Treatments | Collagen Type-II | CSPG | S100 | |||
P-value | Sig diff | P-value | Sig diff | P-value | Sig diff | |
TGFβ1 - TGFβ2+3 | 0.001 | *** | 0.001 | *** | 0.001 | *** |
TGFβ1 - TGFβ1+2+3 | 0.001 | *** | 0.001 | *** | 0.001 | *** |
TGFβ2 - TGFβ3 | 0.002 | ** | 0.266 | 0.005 | * | |
TGFβ2 - TGFβ1+2 | 0.001 | *** | 0.001 | *** | 0.002 | ** |
TGFβ2 - TGFβ1+3 | 0.001 | *** | 0.013 | * | 0.001 | *** |
TGFβ2 - TGFβ2+3 | 0.001 | *** | 0.013 | * | 0.001 | *** |
TGFβ2 - TGFβ1+2+3 | 0.025 | * | 0.002 | ** | 0.338 | |
TGFβ3 - TGFβ1+2 | 0.001 | *** | 0.001 | *** | 0.001 | *** |
TGFβ3 - TGFβ1+3 | 0.005 | * | 0.005 | * | 0.001 | *** |
TGFβ3 - TGFβ2+3 | 0.002 | ** | 0.148 | 0.001 | *** | |
TGFβ3 - TGFβ1+2+3 | 0.001 | *** | 0.025 | * | 0.338 | |
TGFβ1+2 - TGFβ1+3 | 0.001 | *** | 0.001 | *** | 0.002 | ** |
TGFβ1+2 - TGFβ2+3 | 0.001 | *** | 0.001 | *** | 0.001 | *** |
TGFβ1+2 - TGFβ1+2+3 | 0.539 | 0.001 | *** | 0.002 | ** | |
TGFβ1+3 - TGFβ2+3 | 0.148 | 0.148 | 0.001 | *** | ||
TGFβ1+3 - TGFβ1+2+3 | 0.001 | *** | 0.338 | 0.001 | *** | |
TGFβ2+3 - TGFβ1+2+3 | 0.001 | *** | 0.338 | 0.001 | *** |
Conclusion
The expression of Collagen Type-II, S-100 protein, and CSPG was detected through immunocytochemistry of high-density primary chondrocytes cultured in a medium with and without various isomers of TGF-β, including the manipulated versions. It has been previously demonstrated that Smad proteins are critically important for the induction of CSPG production in response to TGF-β. However, there is a differential effect of TGF-β signaling genes, specifically Smad2 and Smad3 [35], with Smad3 reduction showing higher efficacy.
Collagen Type-II expression was increased by manipulated TGF-β1&2&3 and slightly decreased by TGF-β2&3, which is attributed to the influence of TGF-β on ECM production and subsequent cartilage formation. The combination of TGF-β1&2&3 demonstrated an increase in the production of Collagen Type-II. Primary chondrocytes exhibited nuclear staining for CSPG in media with and without TGF-β. Overall, the combination of transforming growth factors reduced the expression of chondroitin sulfate glycoprotein. TGF-β did not induce the expression of S-100 protein, while Collagen Type-II expression was increased by manipulated TGF-β1&2&3 and slightly decreased by TGF-β2&3, which ultimately resulted in ECM production and cartilage formation. Primary chondrocytes displayed nuclear staining for CSPG in media with and without TGF-β. Additionally, the combination of TGF-β reduced the expression of CSPG.
In conclusion, TGF-β did not induce the expression of S-100 protein, and the expression of fibronectin by high-density chondrocytes was not significantly affected by any isomers of TGF-β, including the manipulated forms. However, TGF-β2&3 showed a slight increase in laminin expression. Nevertheless, TGF-β ultimately decreased the synthesis of laminin by primary chondrocytes cultured in the planar system.
Statements and Declarations
Authors' contributions
Hoda Nassira: Project Administration, Conceptualization, Investigation, Data Curation, Writing- Reviewing and Editing, Supervision; Gunay Akbarova: Conceptualization, Investigation; Abolfazl Azadi: Investigation, Writing—Editing; Seyed Ali Behruz Khaghani: Project Administration, Formal Analysis, Visualization, Writing—Original draft, Funding Acquisition, Supervision.
Competing Interests
The authors declare no conflicts of interest.
Ethics Approval
Not applicable.
Data Availability
All data in the paper are available from the corresponding authors upon reasonable request.
Funding
No funding was received to support this work.
Acknowledgments
This work was financially supported by the faculty of Engineering and Informatics and the faculty of Life sciences of the University of Bradford.
Authors’ Information
Hoda Nassira—Polymer Division, Department of Chemistry, Faculty of Science, University of Zanjan, Zanjan 45371-38791, Iran;
Gunay Akbarova—Department of Genetics and Theory of Evolution, Faculty of Biology, Baku State University, Baku, Azerbaijan;
Abolfazl Azadi—Polymer Division, Department of Chemistry, Faculty of Science, University of Zanjan, Zanjan 45371-38791, Iran.
S A Behruz Khaghani—Department of Biomedical and Electronics Engineering, School of Engineering, University of Bradford, Bradford, UK;