Visual Abstract
Abstract
Nucleic acid therapy utilizing ribonucleic acid (RNA) biomacromolecules has attracted considerable attention for being applied as preventive and therapeutic strategies in several diseases including cancer, inflammatory conditions, and neurodegenerative disorders. However, some properties like safety profile, synthesized under good manufacturing procedures, and the fact that RNA molecules, unlike deoxyribonucleic acid (DNA), does not require crossing the nuclear membrane for expression, made them suitable gene materials for being encapsulated in various nano-delivery carriers and delivered precisely to the site of interest. In this article, we summarize the application of organic nano-delivery systems for delivering the two most applied RNA molecules, messenger RNAs (mRNAs) and small interfering RNAs (siRNAs) in different human diseases.
Introduction
Ribonucleic acid (RNA), comprised of nucleotides and existing mostly in the single-stranded form, is an important molecule in the central dogma which is formed from deoxyribonucleic acid (DNA) via the transcription process and is responsible for protein synthesis through the translation process. RNA molecules are present in both eukaryotes and prokaryotes and the complex structural organization of the transcriptome (the RNA structurome) has been known as the key factor for their regulatory function in almost all cellular processes including gene expression [1, 2]. Specific types of RNA molecules could serve as therapeutic agents in human diseases rather than just being involved in cell regulation. Messenger RNAs (mRNA) are the product of DNA transcription and carry the genetic blueprint for protein synthesis. In eukaryotes, the newly transcribed RNA transcript (pre-mRNA) needs to undergo maturation via splicing the non-coding regions (introns) and joining together the coding regions (exons) to form mRNA (Figure 1) [1, 3].
The mRNA molecules have been gaining special attention for therapeutic applications in gene therapy in various health issues since 1990. Different kinds of nucleic acids have been employed in this concept, among them, mRNAs, specifically in vitro transcribed mRNA (IVT mRNA) exhibited vast properties including higher safety performance than DNA for clinical applications due to the controlled expression of specific genes with minimal risk of insertional mutagenesis (since mRNA is translated in the cytoplasm with no integration into the host genome), fast and transient protein expression, scalable manufacturing, and possessing variable functions in medical fields like vaccine production and tissue engineering [4–8].
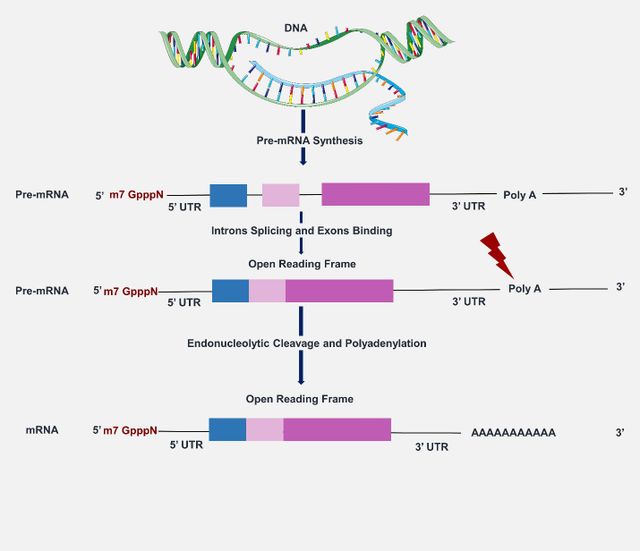
Therefore, mRNA could be considered as a potential novel therapeutic agent that is capable of being used in preclinical and clinical studies to treat many health problems such as infectious diseases, cancers, neurodegenerative disorders, bone degenerative and gastrointestinal diseases, as well as genetic disorders [9–16]. However, mRNA-based therapies still encounter obstacles like optimizing delivery efficiency and precise targeting due to mRNA’s significant sensitivity to the nucleases, low stability, negatively charged, and insufficient available strategies for safe delivery of this important nucleic acid to the site of interest, despite the highlighted potency of mRNA delivery in therapeutic applications including immunotherapy, gene editing, and vaccine productions [17–19]. To overcome these barriers and to enhance the mRNA delivery efficiency for clinical applications, two strategies including mRNA chemical modifications through N6-methyladenosine, 5-methylcytidine, pseu douridine (Ψ), and ribose 2′OMe modifications in addition to utilizing different nano-delivery carriers for transferring mRNA to the targeted site have been developed [20–22].
In recent decades, nanotechnology has been employed in various health issues, clinically for diagnostic imaging and delivery of therapeutic agents (drugs, antibodies, genes, …) to the sites of interest in the body [23]. In this regard, nucleic acid-based vaccines, especially mRNA vaccines have gained considerable attention being employed against human diseases due to their rapid production and the fact that they do not induce genome instability. Nevertheless, there are some challenges to mRNA effective delivery including innate immunogenicity because of the recognition of RNA molecules as a signal of viral infection as well as mRNA sensitivity to nucleases which leads to translation deficiencies [24]. For instance, nanocarrier-based mRNA vaccines are capable of activating the antigen-presenting cells through tumor antigen expression and contribute to innate and adaptive immune activation [25].
Chemically modified mRNAs (cmRNA) have also been investigated for therapeutic applications including bone healing. In a study, bone morphogenetic protein–2 encoded cmRNAs were delivered by nonviral lipid vectors to femoral osteotomies in rat models. The results revealed complete healing of the vast segmental defects at the exact targeted site through the native endochondral route and upregulation of some extracellular matrix components like collagen I and V, osteopontin, osteonectin, and matrix metalloproteinase–2/13 [26].
In addition to various lipid-based nanoparticles, metal-organic frameworks, and polymeric nanocarriers for nucleic acid including mRNA delivery, protein-derived cell-penetrating peptides (CPPs) composed of 4–40 amino acids, and cationic, amphipathic, and hydrophobic peptides have been formulated which can internalize the cell via endocytosis and/ or direct cell membrane penetration. Recorded data highlighted the use of CPPs for mRNA delivery (CPP/mRNA complexes or combined with other nanoparticles) to be effective for stabilizing intracellular mRNAs (cationic CPPs), endosomal pathways modulating to enhance dendritic cells’ antigen-presenting ability, and improving mRNA uptake in lungs through dry powder inhalation [27–29].
Small interfering RNAs (siRNAs), first discovered in 1998 and comprised of 21–25 double-stranded RNA nucleotides, are the main components of RNA interference molecules that activate enzymes like ribonucleases on specific sequences, and post-transcriptionally. RNA interference molecules have also been considered therapeutic agents by inhibiting the expression of DNA into proteins via making bounds to mRNA molecules and directing them to the RNA-induced silencing complex which leads to mRNA cleavage and protein production blockade. The siRNAs are naturally synthesized by Dicer-mediated cleavage of larger double-stranded RNAs and they are capable of silencing the target genes through an RNA interference mechanism which results in gene activity regulation by RNA cleavage or translation repressing (Figure 2) [30, 31].
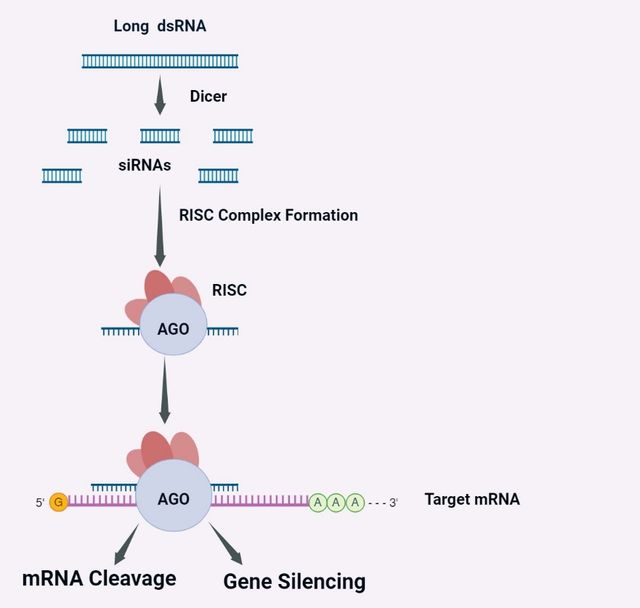
Moreover, they have been known as potential therapeutics for different human diseases including cancer, neurodegenerative disorders, gastrointestinal diseases and inflammation, viral and bacterial infectious conditions, as well as bone and joint disorders attributed to their ability to mediate RNA interference in mammalian cells [32–37]. To improve the challenges in siRNA transfer like biodistribution, delivery specificity, plus immunological responses and to achieve a sustained release, acceptable absorption, reduced drug resistance, and off-target effects, as well as non-toxic activity, different nano-delivery systems have been utilized for siRNA loading to reach a more specific and efficient therapeutic outcome [31, 36, 38].
In this article, we represent the application of organic-based nano-delivery carriers for delivering mRNA and siRNA biomolecules in experimental models of different human diseases.
Application of RNA-loaded Organic-based Nano Vehicles in Different Diseases
One of the major challenges in biological fields of study is the precise delivery of genes and nucleic acids to the site of interest. In this regard, the application of various types of nanoparticles including organic-based vectors has been investigated in several experiments and has revealed promising potency for better cell internalization [39].
Cancer
Cancer is a mortal human health issue around the world, responsible for almost 600, 000 deaths in the United States in 2020, and lung cancer accounts for the first cause of death in the United States among the other cancer types [40, 41]. All cancer types are unified at the cellular phenotype level which is defined as cancer hallmarks that make normal cells capable of turning into neoplasms [42]. Early detection is a crucial factor for improving the survival rate in cancers and despite all advancements, still about 50% of all cancer types remain hard to diagnose at early stages [43]. Treatment options for cancer are almost limited to chemo and radiotherapy plus immunotherapy in which antigen-presenting cells modulate the host’s immune responses through activation of important lymphocytes, but they have been confronting several drawbacks like multi-drug resistance and limited drug accumulation at the tumor site [44, 45]. To overcome these restrictions, nanomedicines (~ 1–100 nm) possessing various physico-chemical properties have been used in cancer therapy. Although nanomedicines have demonstrated effectiveness in the controlled release and improved biodistribution of therapeutic agents, their success in clinical trials remains low [46, 47]. Different nano-delivery vehicles including porous nanocarriers, metal-organic frameworks, biomimetic photocatalysts, and polymeric nanoparticles have been employed for theranostic applications in various cancers, demonstrating considerable potency for efficient delivery of genes and small lipophilic therapeutic agents in cancer pre-clinical investigations due to their specific properties like sustained drug release, stability, ability to load more than one agent at a time, and stimuli-responsiveness [44, 48–51].
In the context of mRNA delivery, mRNA vaccination has demonstrated promising advancements in preclinical and clinical trials, especially for cancer immunotherapy, but there are still main challenges to their successful translation from the laboratory to the market like insufficient intracellular protein expression, antigen loading, and antigen-presenting cells’ maturation for the subsequent immune activation. mRNA vaccines are primarily designed to interfere with the stimulator of interferon genes and tumor-infiltrating lymphocyte pathways to activate more clusters of differentiation 8+ (CD8+) T-cells involved in tumor proliferation hindrance.
Several strategies have been developed to overcome these barriers including mRNA-encapsulation in nanoparticles (lipid-based, polymeric, protein, or peptide derivatives) to enhance stability and modulate immunogenicity [52–55].
Lipid-based nanoparticles like liposomes are important nano-delivery systems that are usually preferred to other nanoparticles mostly due to their interesting properties such as lower side effects, biocompatibility, high loading capacity, and delivery efficiency, as well as easy preparation, and they could be suitable vaccine adjuvant-delivery systems by possessing proper size, surface charge, and membrane flexibility for gene and RNA delivery [56–58]. In a recent study, the application of RNA-embedded multi-lamellar lipid aggregates, using mRNA as a molecular bridge with cationic liposomes, revealed fast reprogramming of the tumor microenvironment in less than 24–48 hours, leading to the effector actions of activated T cells which overcomes the first step necessary for successful cancer immunotherapy. These nanocarriers also improved multiple payload packaging for anti-cancer immunity induction and increased immunogenicity via a multi-lamellar design whereas their cationic charge and intravenous route of delivery modulated the tumor microenvironment in canine glioma subjects, improving their survival rate [59]. Similarly, Metzloff et al. fabricated an activated lipid nano-delivery system, capable of mimicking the activating function of antigen-presenting cells, by using a thiol-maleimide reaction to conjugate CD3 and CD28 antibody fragments to the surface of optimized T-cell lipid nanoparticles. The results showed the potency of these activated lipid nanoparticles to enable one-step activation and transfection of primary human T cells. Moreover, the resulting mRNA chimeric antigen receptor T cells have reduced successfully the tumor burden in a murine xenograft model, confirming these nano-delivery systems, promising for rapid production of mRNA chimeric antigen receptor T cells for cancer immunotherapy [60]. The vaccination of mice models with lipoplexes (cationic liposomes) embedding hybridized mRNA with immunostimulatory short double-stranded RNA also revealed increased antigen-specific cytotoxicity due to the activation of dendritic cells in immune tissues plus a higher level of inflammatory molecules including interleukin–6 and interferon-β in serum [61].
Polymeric-based nano vehicles have also shown significant promise for being used as vaccines and drug/gene delivery systems for cancer therapeutics attributed to their stability, easily functionalized, and modifiable chemical structure which improve the therapeutic efficacy [62, 63]. In a research conducted by Le et al. bevacizumab (an anti-vascular endothelial growth factor antibody)-encoding mRNA molecules were encapsulated in poly (beta-amino esters)-based nanoparticles and then were administered intravenously in orthotopic non-small cell lung carcinoma mouse models. The results revealed a notable distribution of nanoparticles in lung endothelial cells, leading to bevacizumab secretion as well as considerable tumor proliferation and angiogenesis blockade, supporting the therapeutic potential of bevacizumab mRNA therapy and its selective delivery through lung-targeting nanoparticles (Figure 3. A-G) [64].
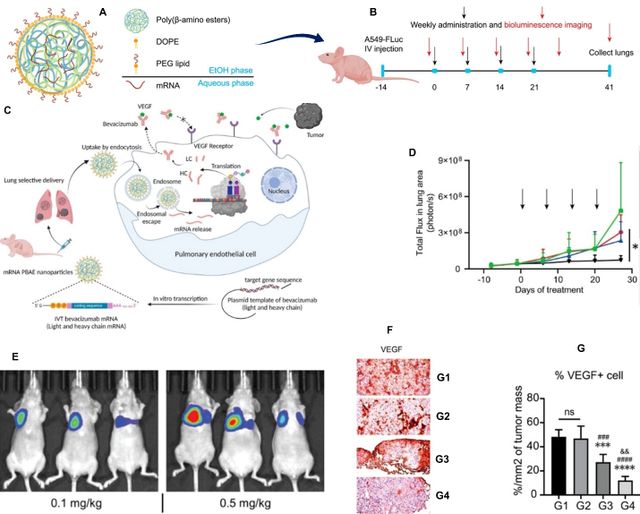
The same nanoparticles, lipophilic poly (beta-amino ester) ones were used in another study to co-deliver mRNA constructs encoding a signal 2 co-stimulatory molecule and a signal 3 immuno-stimulatory cytokine (interleukin–12), along with a nucleic acid-based immunomodulatory adjuvant. Following intratumoral injection of nanoparticles via an injectable thermoresponsive gel, enhanced immunostimulatory cytokine production and immune cell recruitment were achieved which represent these nano carriers’ translational potential as immuno-oncology therapeutics [65].
In bladder cancer mice models, the application of mucoadhesive polyplexes embedding lysine-specific demethylase 6A-mRNA showed increased nanoparticles’ penetration and more controlled release at the bladder tumor site resulting in better metastasis hindrance, as well [66]. Additionally, in their interesting experiment, Parayath et al, employed injectable IVT engineering chimeric antigen receptors expressing mRNA-loaded nanoparticles for temporary reprogramming induction in T lymphocytes and identifying disease-specific receptors. Repeated infusion of these mRNA-loaded vehicles in lymphoma, hepatitis B- induced hepatocellular carcinoma, and bladder cancer in orthotopic xenograft mice models showed anti-cancer responses via genetically reprogramming of T-cells plus increased survival rate (40 days more on average), and a higher rate of T-cells’ trafficking in treatment groups [67]. The mRNA-loaded micelles have also been used as vaccines for immunotherapy treatments. In vivo administration of oligopeptide end-modified poly (β-amino esters)- based mRNA polyplex showed improved cell penetration and antigen-presenting cells targeting for immune response stimulation in addition to the significant uptake of antigen-coding mRNA-encapsulated poly (lactic acid) nanoparticles by dendritic cells through clathrin-dependent endocytosis and phagocytosis, resulting in dendritic cells’ immune responses modulation in vitro, seen in another study [68, 69]. Regarding siRNA delivery for cancer therapy, the application of self-nano emulsifying drug delivery systems, using a chitosan-RNA core, has been investigated for co-delivery of siRNA and hydrophobic chemotherapeutics including doxorubicin, valrubicin, and methotrexate in addition to photosensitizers (rhodamine b and protoporphyrin IX) in colon and breast cancer cell lines as well as in mice models. The results showed a higher rate of siRNA internalization in cancer cells compared to free siRNAs and increased duodenum permeability [70]. Due to the high prevalence of breast cancer, several studies have been performed to investigate the therapeutic potential of siRNA-loaded delivery systems in this disease. For example, in a recent study, the application of siXBP1-embedded poly (lactic-co-glycolic acid)-lipid hybrid nanoparticles, conjugated with an epidermal growth factor receptor antibody, in triple-negative breast cancer in vitro model, contributed to efficient delivery of siRNA to the cancer cells and XBP1 gene (crucial for triple-negative breast cancer progression) silencing, resulting in a threefold higher rate of apoptosis induction under hypoxic conditions. Also, surface engineering of the nanoparticles with epidermal growth factor receptor antibodies improved their precise targeting ability to the tumor sites [71]. Similarly, concurrent delivery of paclitaxel and AXL gene targeting siRNA (siAXL) by Poly (ethylene glycol) monomethyl ether (mPEG)-camouflaged dendritic polylysine nanocarriers showed enhanced cell uptake, tumor targeting, and permeability of nanoparticles in vivo triple-negative breast cancer model. Additionally, in vitro investigations revealed increased paclitaxel’s toxicity, apoptosis, and cell cycle arrest efficiency as well as its sensitization to siAXL [72]. In the context of siRNA delivery for other types of cancer, Lin et al. demonstrated that following the application of CD 47 targeting siRNA-loaded poly (ethylene glycol)-b-poly (D, L-lactide)-cationic lipid-ionizable lipid delivery system, a significant increase in the siRNA silencing efficiency and immune response induction occur due to the presence of ionizable lipids. This strong immune response suppresses the proliferation of melanoma tumors via inhibiting CD 47, a crucial immune checkpoint, leading to the introduction of this interesting formulation as a novel siRNA delivery system for gene silencing and cancer immunotherapy [73]. In addition, combinational delivery of cisplatin and siRNA against HPV 16 E6/E7 by engineered ionizable lipid nanoparticles (ENB101-LNPs) revealed considerable tumor cell growth hindrance and apoptosis induction in both in vitro and in vivo models of cervical cancer [74]. The plasmid clustered regularly interspaced short palindromic repeats (p CRISPR) has also shown promising cancer-fighting properties while co-delivered with doxorubicin to different cancer cell lines by a novel formulated nanocomposite composed of amine-functionalized metal-organic frameworks, conjugated to poly (aniline-co-para-phenylenediamine), and coated on manganese ferrite nanoparticles. The results indicated
successful delivery of p CRISPR and doxorubicin to the cancer cells, introducing this delivery system promising for multi-drug delivery according to its crucial characteristics including biosafety resulting from its hemocompatibility profiles (less than 1% hemolysis), increased cellular uptake (up to 38.3% in A549 cells), improving transfection, and acceptable delivery and expression in a physiological microenvironment [75].
Aging and Age-related Diseases
Several studies have revealed the crucial role of oxidative stress, excessive production of reactive oxygen species by the body under abnormal physiological conditions, in pathological changes in cells including DNA damage, mitochondrial dysfunction, telomere shortening, and protein oxidative modification, leading to apoptosis induction, aging, and age-related issues like retinal diseases, neurodegenerative disorders, hypertension, abdominal aortic aneurysms, atherosclerosis, cancer, osteoarthritis, ovarian and prostate diseases, as well as type II diabetes [76–79]. To improve the therapeutic outcomes of age-related conditions, the application of different RNA delivery systems such as extracellular vesicles has demonstrated promising potencies and RNA-based vaccines have also proven to be capable of targeting specific antigens and inducing immune responses [76]. In the context of skin aging therapy, application of glutathione S-transferase M2 mRNA-embedded skin primary fibroblasts-derived extracellular vesicles showed significant improvement in skin homeostasis and wound healing in aged mice models through mitochondrial oxidative phosphorylation modulation, reduction of oxidative stress damage of aging dermal fibroblasts, and regulation of the skin epidermal cell function by paracrine secretion of nascent polypeptide-associated complex alpha subunit, confirming these delivery system promising for aged skin treatment [80]. Similarly, Chang et al. have demonstrated that human telomerase reverses transcriptase mRNA-loaded lipid nanoparticles are capable of accelerating the delivery and expression of mRNA in vitro in keratinocytes, fibroblasts, and in human-skin-cell-suspension (hSCS) prepared from donors’ skin, resulting in enhanced telomerase activity, hSCS cellular engraftment and proliferation, partial-thickness human skin equivalent in the mouse model generation, in addition to cellular senescence and DNA damage reduction. Together, the results of this interesting research work introduce these mRNA delivery systems as novel therapeutic strategies for wound healing [81]. Regarding siRNA therapy for age-related diseases, cartilage affinity peptide-surface engineered exosomes, encapsulating matrix metallopeptidase–13 targeting siRNAs have been investigated in rat models of anterior cruciate ligament transection-induced osteoarthritis. The intra-articular administration of exosomes resulted in matrix metallopeptidase–13 level reduction plus the cartilage collagen (COL2A1) and proteoglycan enhancement via precise targeted delivery of siRNA to chondrocytes, leading to cartilage degeneration alleviation [82].
Alzheimer’s Disease
Alzheimer’s disease is a progressive neurodegenerative disorder, known as the most prevalent cause of dementia in the elderly, representing memory loss with varying cognitive dysfunctions, with no effective treatment to target the main route responsible for its pathology in the brain. Several factors including genetics, aging, and environment are mentioned as its etiology, and to date, various hypotheses like the cholinergic, amyloid-beta plaques and tau neurofibrillary tangles, inflammatory, oxidative stress, metal ion, glutamate excitotoxicity, microbiota-gut-brain axis, and abnormal autophagy have been discussed as its pathophysiology [83, 84]. The main reasons for unsuccessful drug delivery to the brain are the blood-brain barrier and the blood-cerebrospinal fluid barrier which act as obstacles. So, the need for the application of nanotechnologies to specific brain targeting and improve brain bioavailability has been highlighted and various nanocarriers have been applied for drug delivery in Alzheimer’s disease treatment, in this regard [85] [86]. In a pilot study for developing an mRNA-loaded lipid-based Alzheimer’s disease vaccine, targeting the N-terminus of pathological amyloid-beta in mice models and non-human primates, researchers found that developing a preventive vaccine through this strategy could stimulate long-lasting antibodies in individuals at risk of Alzheimer’s disease through inhibiting the aggregation of pathological amyloid-beta and tau proteins as well as delaying the onset of dementia [87]. Also, Lin et al. evaluated the ability of neprilysin expressing mRNA-loaded polyplex nano micelles for degradation of amyloid-beta in the mouse brain via intracerebroventricular infusion to the 3rd ventricle. The results revealed a considerable attenuation of amyloid-beta 40 concentration and the neprilysin expression augmentation in the brain tissue [88]. In the context of siRNA therapy, co-delivery of β-site amyloid precursor protein cleaving enzyme–1 siRNA plus donepezil, and memantine with liposomes for Alzheimer’s disease therapy have also been investigated, in vitro. The therapeutic agents including siRNA were effectively delivered intracellularly and the fabricated liposomes showed the potential to overcome tight junctions in the blood-brain barrier in addition to reducing amyloid-beta plaque accumulation and pro-inflammatory cytokine expression [89]. Furthermore, cyclin-dependent kinase inhibitor 2A (p16ink4a)-siRNA-loaded poly (D, L-lactic-co-glycolic acid) (PLGA) nanoparticles showed enhanced p16ink4a expression in microglia near the amyloid plaques in brain tissue from Alzheimer’s disease patients and mice models and microglia with enhanced amyloid phagocytic capacity transformation via cell cycle regulation as well as cell growth augmentation were also observed. Together, nanoparticle-based delivery of p16ink4a siRNA contributed to a significant decrease in the number of aged microglia surrounding the plaques and amyloid plaque formation, leading to reversed learning destruction and spatial memory deficits [90].
brain disorders
Despite all advancements in the treatment of neuropathological conditions like brain tumors, efficient delivery of therapeutic agents remains unsuccessful due to the presence and traffic regulatory profile of three main physiological barriers including the blood-brain barrier and the blood-leptomeningeal barrier between the central nervous system and the bloodstream, plus blood-cerebrospinal fluid barrier between choroid plexus stroma and cerebrospinal fluid inside the ventricles [91]. Among these barriers, the blood-brain barrier plays a crucial role in impeding the delivery of therapeutic molecules from blood to the brain and its malfunction is responsible for almost all brain pathological conditions. However, the existence of different receptors and transporters on the blood-brain barrier enables brain-targeting drug delivery via receptor-mediated transcytosis and carrier-mediated transportation mechanisms [92]. Although the application of different nanotechnology-based strategies for efficient brain delivery has been growing in recent decades, still there is no FDA-approved nano-delivery system for brain disorders mostly due to the brain tissue vulnerability, intricacy of the disease nature, plus physiological barriers. Nevertheless, nanoparticles’ parameters especially their composition are proved to have important effects on drug transportation through the blood-brain barrier [93]. Studies demonstrated that some strategies including nanoparticles’ size control, surface functionalization, and co-delivery of mucolytic agents result in the prevention of the bio-nano interactions between pulmonary delivered nanocarriers and the physiological barriers which lead to inhibition of the nanoparticles’ effective release in the targeted tissue [94]. To date, different nanoformulations including liposomes and nano micelles have been employed for improved transportation of the therapeutic agents through the blood-brain barrier [95]. In a study, Abbasi et al. investigated the genome editor properties of CRISPR-associated protein 9 (cas9) mRNA and single guide RNA-loaded nano micelles in mice brains. In this experiment, intraparenchymal injection of polyplex nano micelle in mice’s cerebral cortex resulted in enhanced genome editing in mouse brain parenchymal cells like astrocytes and microglia plus a higher rate of nano micelles’ dissemination in the brain tissue [96]. Furthermore, the application of polyethylene glycol-coated polyplex micelles, encapsulating bundled mRNA which was made through the hybridization of mRNA strands with RNA oligonucleotide cross-linkers, represented increased nano micelles’ stability against ribonuclease activity and polyion exchange reaction plus improved mouse blood circulation stability [97].
Gastrointestinal Diseases
Various factors including environmental conditions, genetic alterations, and the interactions between the gut bacterium and the host’s immune system are responsible for pathologies like inflammatory bowel disease (IBD) in the human gastrointestinal pathway. Due to the side effects accompanied by the consumption of anti-inflammatory and immune suppressive medications including toxicity issues and insufficient drug delivery to the target sites, nanotechnology-based therapies have been applied for more precise drug transmission with the lowest rate of systematic injuries [98, 99]. To evaluate the efficacy of mRNA delivery systems for improved treatment of gastrointestinal disorders, a study by Patel et al. has investigated the effects of incorporating bile acids, instead of cholesterol, into a series of lipid nanoparticle formulations on the biodistribution and relative expression of mRNA cargo in several organs including liver, spleen, kidneys, small and large intestine, and stomach of mice models following intravenous or intraperitoneal injection. The results showed that these bile-acid-containing lipid nanoparticles reduce delivery to liver cells in vitro and improve delivery in various other cell types, including T cells, B cells, and epithelial cells. Of note, substituting cholic acid for cholesterol in a lipid nanoparticle containing an ionizable lipid, C12–200 containing Cholic Acid–100, shifts the mRNA delivery from the liver to the spleen (greater spleen mRNA expression than liver mRNA expression, indicating primarily spleen delivery), suggesting that this cholic acid replacement strategy could be promising for an extrahepatic mRNA therapy for improved gastrointestinal or immune cell delivery in different health issues including gastrointestinal disorders [100]. Regarding IBD treatment, the application of mRNA–1273 vaccine in tumor necrosis factor inhibitor-treated IBD patients who have had an experience of SARS-CoV–2, revealed significant induction of spike-specific CD8+ T cell responses with a predominant central memory and activated phenotype, making this vaccination suitable as a potential therapy for this disease [15]. Moreover, polymer and dendrimer modified-small-sized graphene oxide nanoparticles embedding tumor necrosis factor-α siRNA have been fabricated and utilized for investigating their therapeutic potency in organotypic mouse models of chronic inflammation in small and large intestines, induced by inflammatory agents interleukin–1β, tumor necrosis factor-α, and lipopolysaccharide. The results demonstrated that the high transfection efficiency of these nano vehicles leads to the downregulation of pro-inflammatory cytokines and inflammation reduction [101]. Also, Chen et al. formulated a polyphenol-based nanosystem to be administered via rectal in dextran sulfate sodium salt-induced acute and chronic ulcerative colitis mice models. The results revealed interleukin–10 upregulation and inflammatory agents’ expression hindrance, plus improved protection of colonic epithelial cells against apoptosis which makes this nanoformulation potential for ulcerative colitis treatment via mRNA delivery [102].
Conclusion and Future Prospects
Nucleic acid-based therapies, especially mRNAs and siRNAs, have been gaining considerable attention in biomedical fields of science and for the treatment of a wide range of human health problems including neurodegenerative disorders, inflammations, cancers, etc. However, attributed to the immunogenicity, high sensitivity to nuclease enzymes, and low half-life of RNA molecules, different nanotechnology-based strategies like polymeric and lipid-based nanoparticles have been utilized for their encapsulation to achieve precise targeting, prolonged circulation time, and controlled release.
However, to realize the potency of RNA delivery by various nanosystems, more investigation is needed to correlate the findings in the laboratory to clinical trials since despite all advancements in RNA-based therapies, specifically for vaccine developments, the production processes are still limited to bench-scale methods rather than being suitable for clinical applications and this is one of the main challenges in this field. Nevertheless, some key factors like employing representative animal models for preclinical evaluations, intellectual drug delivery design, and having specific protocols to be applied in clinical trials could be effective in proceeding with the nanotechnology-based research to the clinical administration. According to the limitations of nomedicine development and application in various health issues, it is essential to have a good knowledge of the main barriers and future demands to attain better results.
Statements and Declarations
Authors’ Contributions
Conceptualization and Writing—Original Draft: M Motallebi; Writing—Review & Editing: M Motallebi; S Malyen; G Ao
Competing Interests
The authors declare no competing interests.
Ethics Approval
Not Applicable.
Data Availability
Not Applicable.
Funding
This article has received no funding.
Authors’ Information
Mahzad Motallebi—Nanomedicine Research Association (NRA), Universal Scientific Education and Research Network (USERN), Tehran 7616911319, Iran;
Samira Malyen—Nasibeh School of Nursing and Midwifery, Mazandaran University of Medical Sciences, Sari, Iran;
Geriletu Ao—The Research and Development Center of endoscopic instruments engineering in Zhejiang province, The Quzhou Affiliated Hospital of Wenzhou Medical University, Quzhou People’s Hospital, Quzhou 324000, Zhejiang Province, China;