Visual Abstract
Abstract
The increasing use of natural and biodegradable polymers, particularly cellulose and its derivatives in food industry to replace petroleum-based plastics is demanded. Cellulose is abundant, cost-effective, and it is sourced from bio-wastes and agricultural wastes that makes it a sustainable alternative. Despite its promising mechanical and barrier properties, cellulose high hydrophilicity limits its use. Chemical modification can enhance its mechanical property, thermal stability, and biodegradability those recommend cellulose for food industry as raw food materials, additive ingredients, packaging materials, delivery system, and for enzyme and cell immobilization. This review covers the significance, function, and attributes of cellulose and its derivatives in food industry.
Introduction
Due to the rising requests for maintainable options to reduce the negative environmental impacts of regular materials in the food industry, the worldwide food industry has been experiencing a significant attempt for a long time. Examining renewable and biodegradable possibilities is essential in developing concerns about plastic contamination, the carbon effect on the food industry, and the resource limit [1]. The most prevalent natural polysaccharide in almost all plant materials is cellulose. Cellulose is safe for human health, biodegradable, and non-toxic, and recommended for widespread usage in the food sector as dietary fiber [2].
Since the beginning of the twenty-first century, research on edible films and active packaging manufactured with cellulose and its derivatives has been primarily patent-protected for use it in the food area [3]. Numerous works have been established to obtain micro and nanocellulose from diverse cell categories [4]. The need for this particular cellulose in the food industry is further increased by its use in various foods such as liquid, syrup, fatty, and semi-fatty categories as flavorings, stabilizers, and thickeners. These indigestible and insoluble fibers have certain physical properties that enhance the product’s quality, safety, and structure. The use of cellulose in the form of fibers is increasing yearly due to its availability, efficiency, variety, and affordability in function [2]. For example, methylcellulose (MC) and carboxymethylcellulose (CMC) are two cellulose derivatives that are used in the food industry [5,6]. Cellulose and its derivatives will continue to receive the interest of industry and science for use in recycled, sustainable, and biodegradable polymer applications in the food industry.
One of the key points of interest of cellulose in the food industry is its biodegradability. Unlike conventional petroleum-based plastics which remain in the environment for centuries, cellulose can be normally degraded by microorganisms returning to the soil and marine without hazardous debris [7]. Cellulose with functional properties can supply the food industry against humidity, oxygen, and other outside components protecting the freshness and quality of foods [8]. By coordinating cellulose materials in food, the food industry does not address current natural challenges, moreover, it meets the customer’s desire with more eco-friendly consumable items. The diverse applications of cellulose-based materials in the food industry impress their versatility and adequacy and promote food quality and sustainability [9,10].
The subject of the present review is precisely designed to provide a comprehensive understanding of the role and applications of cellulose and its derivatives in food products. The review content typically begins with an introduction to cellulose, a naturally occurring polymer, explaining its structure, sources, and basic properties. This foundational knowledge is essential as it sets the stage for understanding how cellulose is modified to create various derivatives such as cellulose ethers, esters, and microcrystalline cellulose, each possessing unique properties suitable for specific applications in the food industry. The subject then investigates the functional properties of these cellulose derivatives such as their ability to act as thickeners, stabilizers, emulsifiers, and fat replacers. These functionalities are crucial in food processing and product development where texture, stability, and consistency are key quality attributes. The course explores cellulose's chemical modifications to achieve these functionalities and link the molecular structure to its practical applications in foods. Moreover, the subject examines the regulatory aspects and safety concerns associated with the use of cellulose derivatives in food products. Understanding the legal framework and safety guidelines ensures that students or professionals are aware of the compliance requirements in different regions which is essential for the development of safe and marketable food products. The review might also cover current trends and innovations in the use of cellulose and its derivatives, highlighting ongoing research and potential future applications in the food industry.
Properties and reactivity of cellulose
Cellulose is a high molecular weight, linear biopolymer composed of repeating D-glucose units linked by β1→4 glycosidic bonds. One end of the cellulose chain features a hemiacetal group. The structure of cellulose is complex, characterized by various packing and aggregation patterns, which differ among organisms that produce it. Each glucose unit in the cellulose molecule has three hydroxyl groups, which are crucial for forming intra-chain hydrogen bonds. These bonds occur between the hydroxyl groups and the oxygen atoms of adjacent glucose rings, stabilizing the structure and resulting in a linear, unbranched chain configuration [11].
The degree of polymerization (DP) in cellulose, which refers to the number of glucose units in the chain, ranges from 1,000 to 15,000, depending on the source and treatment of the cellulose. This variation significantly influences the properties and applications of cellulose. In nature, cellulose molecules organize into elementary fibrils found in the cell walls of plants, algae, tunicates, or produced by bacteria. These fibrils form ordered, parallel layers, tightly packed together, maintained by van der Waals forces and strong hydrogen bonds, both intra- and intermolecular. The lateral dimension of these fibrils typically ranges from 3–5 nanometers, with each fibril composed of crystalline cellulosic structures interspersed with less ordered, amorphous regions. When these elementary fibrils aggregate further, they form microfibrils, which have a cross-sectional width of 5 to 20 nanometers and can extend several micrometers in length, depending on their biological origin. Cellulose is a semi-crystalline material, containing both crystalline and amorphous regions. The degree of crystallinity—how much of the cellulose structure is ordered versus disordered—varies based on its source, extraction method, and pretreatments. Cellulose derived from wood or plants typically shows a crystallinity of 40–60%, while cellulose from bacteria or tunicates can exhibit much higher crystallinity, ranging from 80% to 100% [11].
In addition to its structural properties, cellulose is notable for its chemical reactivity, primarily due to the presence of three hydroxyl groups in each glucose residue, located at the C2, C3, and C6 positions. These hydroxyl groups exhibit distinct chemical behaviors, with those at C2 and C3 functioning as secondary alcohols and the group at C6 as a primary alcohol. The reactivity of cellulose hydroxyl groups is influenced by their intrinsic chemical properties, steric effects from reacting agents, and steric constraints from the cellulose supramolecular structure. The hydroxyl group at C6 is generally the most reactive due to its primary alcohol nature, followed by the C2 and then the C3 hydroxyl group. This hierarchy in reactivity, expressed as OH–C6 > OH–C2 > OH–C3, is crucial for understanding and predicting the outcomes of various chemical modifications. The degree of substitution (DS) is a critical parameter reflecting the average number of hydroxyl groups per anhydroglucose unit in cellulose that have been replaced or modified in a reaction. Theoretically, the DS for cellulose is three, corresponding to the three hydroxyl groups present in each glucose unit. However, cellulose reactivity is more complex than that of simple trihydric alcohols or sugars due to its high molar mass and fiber-forming nature. Chemical modifications such as esterification, etherification, and graft polymerization, often target these hydroxyl groups to alter cellulose's physical and chemical properties, enhancing its functionality for specific applications. These modifications are pivotal for its use in various industries, including the food sector. The crystalline form of cellulose can exist in several allomorphs, designated as cellulose I, II, III, and IV. Each of these forms has distinct structural characteristics and properties. Refer to (Figure 1) for the molecular structure of cellulose, its hydrogen bond network, and the steps to obtain its polymorphs [11,12].
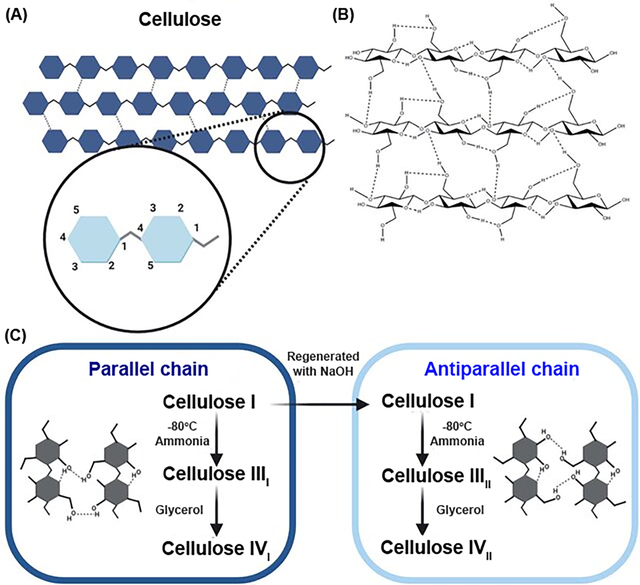
Recent studies have further detailed the variations in hydrogen bonding and conformational arrangements within the Iβ crystal structure of cellulose. Recent studies highlight variations in hydrogen bonding within the Iβ crystal structure of cellulose (Figure 2) [12,13].
This hierarchy in reactivity is essential for understanding and predicting the outcomes of various chemical modifications of cellulose, which are pivotal for its application in different industries, including the food sector. Chemical modifications such as esterification, etherification, and grafting typically target these hydroxyl groups to alter the physical and chemical properties of cellulose, enhancing its functionality for specific applications (Figure 3) [14].
Cellulose sources
Cellulose is a homopolymer consisting of β-D-glucopyranose units linked by (1,4)-glycosidic bonds, making up approximately 40–50% of the dry weight of lignocellulosic materials. The cellulose content varies depending on the type of biomass, growth conditions, and specific parts of the plant. Primary sources of cellulose include wood, non-wood plants, marine organisms, and bacteria, each offering unique properties and advantages for different applications [15].
Wood is the main source of cellulose, typically divided into hardwood and softwood. Hardwood contains about 33–42% cellulose, while softwood has a higher content of 38–51%. The structural differences between these types affect the extraction and quality of cellulose obtained from them.
Non-wood plants such as cotton, bamboo, and agricultural residues like rice straw and sugarcane bagasse are also important sources. Non-wood cellulose is preferred for its lower lignin content, shorter harvest cycles, and reduced environmental impact. These sources are more sustainable and require less energy for cellulose extraction compared to wood [15].
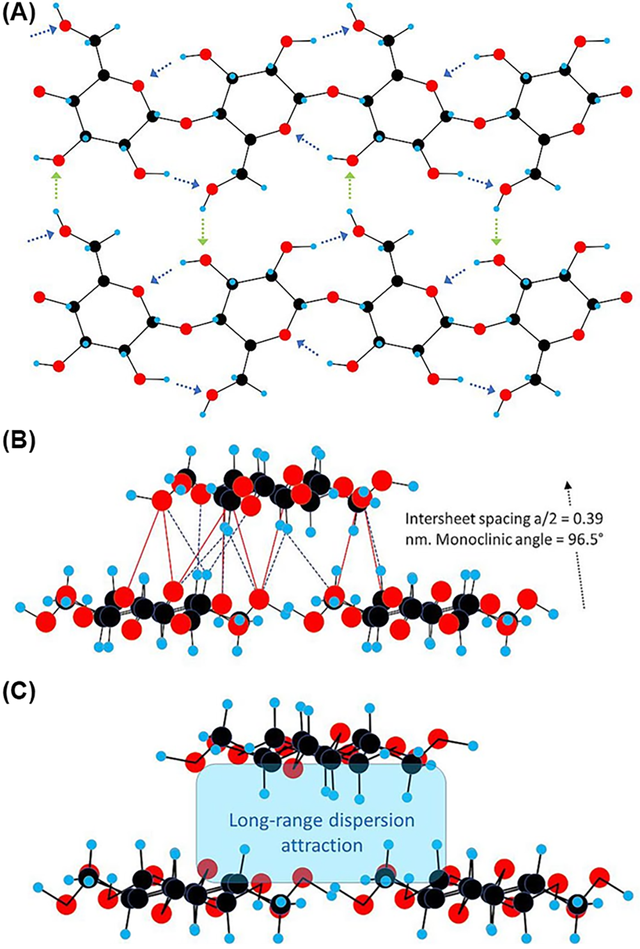
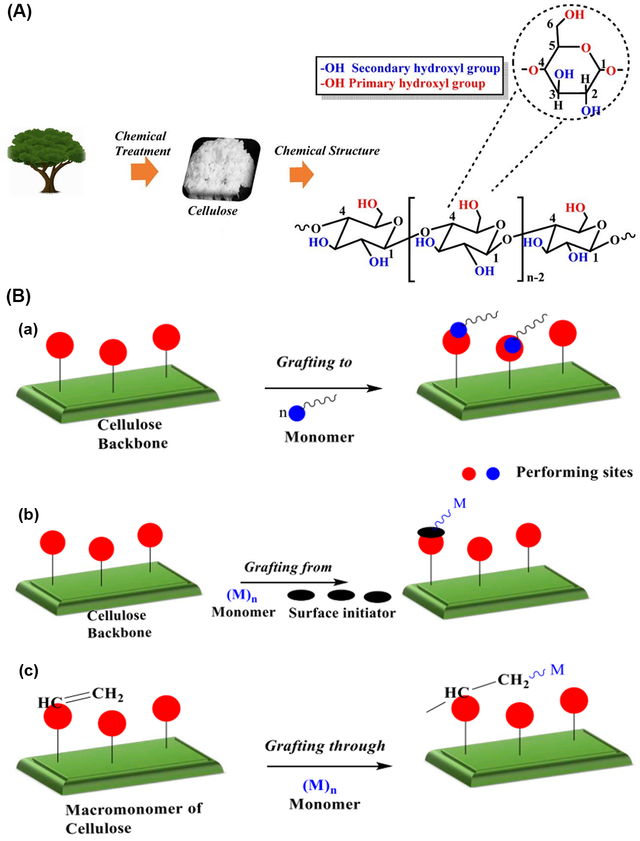
Marine organisms, like tunicates, produce high-purity cellulose known as tunicin, which has a distinct braided structure. Algae such as Cladophora and Rhizoclonium are also rich in cellulose, characterized by high crystallinity and the absence of lignin, making their cellulose easier to extract and purify. Bacteria such as Acetobacter produce bacterial cellulose with high crystallinity and excellent water absorbency. This type of cellulose is free from non-cellulosic impurities, making it suitable for high-value applications, particularly in fields requiring high purity and specific material properties. Extraction of cellulose involves removing lignin and hemicellulose through processes like alkaline extraction and bleaching. The complexity of the extraction process varies with the source material. Plant-based cellulose often requires significant processing, while cellulose from marine organisms and bacteria generally has fewer impurities and requires less purification [16,17]. Different cellulose-based and cellulose content are shown in Table 1.
Cellulose content based on their sources | ||
Wood Cellulose Source | Cellulose Content (%) | Reference |
Hard wood | ||
Acacia mangium | 44.69 | [18] |
Populus sp. | 48.6 | [19] |
Cerasus avium L. | 46.64–48.66 | [20] |
Eucalyptus sp. | 84 | [21] |
Oak | 40.4 | [22] |
Eucalyptus | 40–45 | [23] |
Acacia | 40–45 | [24] |
Soft wood | ||
Pinus merkusii | 43.74–44.8 | [19] |
Picea sp. | 41.9–94.6 | [25] |
Pine | 42–50 | [26] |
Douglas fir | 40–50 | [27] |
Non-Wood Cellulose Source | ||
Sugarcane | 36.81–52.45 | [28] |
Whitened sugar cane bagasse | 77.6–89.3 | [29] |
Empty fruit bunches from Palm oil | 48.92–53.70 | [30] |
Paper | 85–99 | [31] |
Wood bark | 20–30 | [32] |
Bacteria | 20–30 | [32] |
Boehmeria nivea | 69–90 | [32] |
Cotton | 89–93 | [33] |
Ramie seed fibers | 72.1 | [34] |
Pineapple | 73.4 | [35] |
Bean hull | 51.87 | [36] |
Coconut | 32–43 | [37] |
Waste products from the paper/pulp industry | 76.34 | [38] |
Alang-alan pulp | 47.05 | [39] |
Agriculture Waste | ||
Wheat straw | 35-39 | [40] |
Barley hull | 36-43 | [36] |
Rice straw | 29.2-34.7 | [41] |
Rice husks | 28.7-35.6 | [38] |
Oat straw | 31-35 | [42] |
Corn cobs | 33.7-41.2 | [43] |
Sugarcane bagasse | 25-45 | [28] |
Sorghum straw | 32-35 | [44] |
Grasses | ||
Grasses | 25-40 | [45] |
Switchgrass | 35-40 | [45] |
Derivative | Example | Functional group |
Cellulose esters Organic esters | Cellulose acetate Cellulose triacetate Cellulose propionate Cellulose acetate propionate Cellulose acetate butyrate | -CH3(C = O) -CH3(C = O) -CH3CH2(C = O) -CH3CH2(C = O) -CH3CH2CH2(C = O) |
Inorganic esters | Cellulose nitrate Cellulose sulfate | -NO2 -SO3H |
Cellulose ethers Alkyl | Methylcellulose Ethylcellulose Methyl-ethyl cellulose | -CH3 -CH3CH2 -CH3CH2 |
Hydroxyalkyl | Hydroxy-ethyl cellulose Hydroxypropyl cellulose Hydroxy ethyl-methyl cellulose Hydroxypropyl methylcellulose Ethyl hydroxyethyl cellulose | -CH2CH2OH -CH2CH(OH)CH3 -CH2CH2OH -CH2CH(OH)CH3 -CH2CH2OH |
Carboxyalkyl | Carboxymethyl cellulose | -CH2COOH |
Types of cellulose derivatives
Cellulose, a natural polymer, is highly valued for its versatility and ability to undergo various chemical and physical modifications. These modifications enhance its properties and make it suitable for a wide range of applications, particularly in the food industry. Notably, several cellulose derivatives have been developed (Table 2), each characterized by distinct features and specific uses [46].
Methyl Cellulose (MC):
MC is a cellulose derivative produced by replacing the hydroxyl groups in cellulose with methoxyl groups using methylating agents like methyl iodide or chloride in an alkaline medium. This modification gives MC properties such as high viscosity, non-toxicity, and the ability to form tough, durable films. It is widely used in food as a thickener and emulsifier in products like desserts and meat analogs. In pharmaceuticals, MC is a key ingredient in bulk-forming laxatives for constipation and eye drops for dry eyes. Industrially, MC acts as a binder in leather, textiles, and paper, enhancing their durability and quality. It is widely used in food as a thickener and emulsifier in products like sauces, soups, desserts, bread, fried foods, reduced-fat foods, gluten-free bakery products, and meat analogs [46,48].
Hydroxypropyl Methyl Cellulose (HPMC):
HPMC is formed by replacing the hydroxyl groups on cellulose with methoxyl and hydroxypropyl groups. This modification enhances its water retention, gelling properties, and stability in cold and hot water solutions. HPMC is widely used in the food industry for its ability to form stable, high-viscosity solutions that resist enzymatic degradation. It is used in products like whipped toppings, mousses, frozen desserts, dressings, sauces, gluten-free bakery products, and reduced-fat foods [46].
Carboxymethyl Cellulose (CMC):
Also known as cellulose gum, CMC is produced by binding carboxymethyl groups to the cellulose backbone. This derivative is highly soluble in both hot and cold water and is used in the food industry for its ability to improve the texture and stability of products. CMC is often used as a thickener and stabilizer in various food and pharmaceutical applications [49].
Croscarmellose Sodium (CCS):
CCS, a water-soluble form of sodium carboxymethyl cellulose, is widely used across various industries. In pharmaceuticals, it serves as a disintegrant in tablets and capsules, aiding in drug release. In the food industry, CCS acts as a thickener for products like frozen desserts, ice cream, yogurt, dressings, sauces, syrups, beverages, and reduced-fat foods. It is also used in laxatives, textiles, and heat packs due to its high viscosity pdf4, application. Additionally, CCS functions as a binder in battery electrodes, an additive in papermaking, and a component in synthetic detergents, highlighting its versatility and essential role in numerous applications [46,48].
Ethyl Cellulose (EC):
EC is a derivative of cellulose produced by replacing its hydroxyl groups with ethoxy groups through a reaction with ethyl chloride in an alkaline solution. This process involves dispersing cellulose in an aqueous sodium hydroxide solution to form alkali cellulose, which then reacts with ethyl chloride to yield ethyl cellulose. EC is insoluble in water but can dissolve in various organic solvents. Its excellent barrier properties against moisture and oxygen make it highly valuable in multiple applications. In the food industry, EC is used for packaging to protect products from environmental factors. In pharmaceuticals, it serves as a coating agent for controlled drug release, ensuring that medications are delivered effectively over time. Additionally, EC is employed as a binder and stabilizer in a variety of applications, including textiles, inks, and leather, due to its ability to enhance durability and stability [46,48].
Hydroxyethyl Cellulose (HEC):
HEC is created by reacting cellulose with ethylene oxide. It is highly soluble in water across a wide temperature range and is used as a thickening and stabilizing agent in various food products and personal care items [46,48].
Hydroxypropyl Cellulose (HPC):
This derivative is produced by reacting cellulose with propylene oxide. HPC is more hydrophobic and plastic than other cellulose ethers, making it suitable for applications requiring a balance of water solubility and organic solvent compatibility. It is often used in coatings and films for its flexibility and stability [46,48].
Microcrystalline Cellulose (MCC):
MCC is obtained through high-shear treatment of cellulose, resulting in a highly purified, colloidal form. It is used extensively as a filler and binder in tablet formulations, providing structural integrity and stability without dissolving in water. In the food industry, MCC is utilized in products like dressing, beverages, whipped toppings, reduced-fat foods, and ice cream [46,48].
Polyanionic Cellulose (PAC):
PAC is produced by etherifying cellulose with chloroacetic acid in the presence of an alkalized solution. This derivative is highly soluble in both hot and cold water and is known for its excellent thermal stability and salt resistance, making it useful in a variety of industrial applications [46,48].
Hydroxypropyl Methyl Cellulose Phthalate (HPMC-P):
This esterified form of HPMC is produced using phthalic anhydride. It has specific solubility properties depending on the pH level, making it ideal for controlled-release formulations in pharmaceuticals [46,48].
Hydroxypropyl Methyl Cellulose Acetate Succinate (HPMCAS):
HPMCAS is synthesized by esterifying HPMC with acetic and succinic anhydrides. This derivative dissolves at specific pH levels, providing targeted-release properties for active ingredients in food and pharmaceutical products [46,48].
Powdered Cellulose (PC):
Produced through mechanical micro pulverization, PC has high amorphous content and is used as a filler and binder in tablet production. It is not soluble in water or organic solvents, making it useful for structural applications in various formulations. In the food industry, PC is utilized in products like bread, beef burgers, doughnuts, pasta, imitation cheese, and cereal [46,48].
Carboxymethyl hydroxyethyl Cellulose (CMHEC):
Combining the properties of CMC and hydroxyethyl Cellulose (HEC) offers high water retention and compatibility with multivalent cations, enhancing the viscosity and stability of solutions.
These cellulose derivatives demonstrate the versatility and importance of cellulose modifications in enhancing the functionality and applicability of this natural polymer in the food and pharmaceutical industries [46]. Figure 5. represents the cellulose derivatives and properties in the food industry.
Cellulose Solubility and Toxicity
A significant challenge in utilizing cellulose for food industry applications is its inherent insolubility in water and many common solvents. This insolubility is due to the strong intra- and intermolecular hydrogen bonds, as well as electrostatic and hydrophobic interactions within the cellulose fibrils, creating tightly integrated and stable structures that resist dissolution. As a result, the strong bonding within the cellulose chains makes it difficult to dissolve in typical solvents, posing a challenge for its use in food formulations that require soluble or easily dispersible ingredients. To address these challenges, researchers have developed various solvents and modification techniques to improve the solubility of cellulose. Ionic liquids such as 1-butyl–3-methylimidazolium formate (BMIMFmO) and 1-butyl–3-methylimidazolium chloride (BmimCl), have shown promise due to their ability to disrupt the cellulose structure effectively. These solvents are environmentally friendly and offer low toxicity, thermal stability, and recyclability, making them suitable for food industry applications where safety and sustainability are crucial [15].
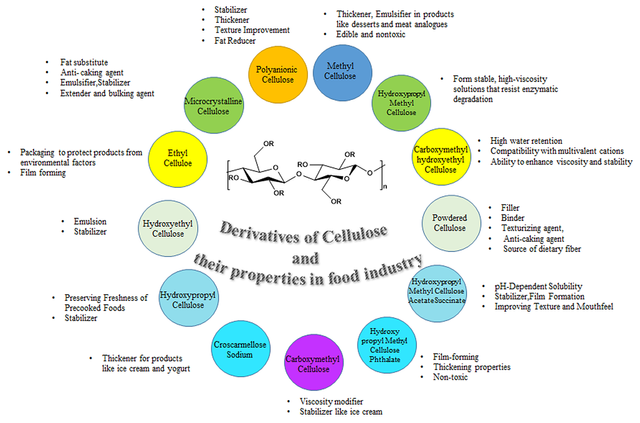
In addition to enhancing solubility, cellulose's impressive mechanical properties make it a valuable ingredient in food industry applications. When correctly modified, cellulose derivatives can provide desirable textural properties such as thickening, gelling, and stabilizing, which are essential for various food products. Crystalline cellulose such as cellulose I, exhibits mechanical properties comparable to high-strength materials, making it useful for creating food packaging materials that require durability and stability. Bacterial cellulose (BC) is particularly noteworthy for its exceptional mechanical properties and has been explored for use in food packaging due to its biodegradability and barrier properties [15]. However, cellulose hygroscopic nature poses challenges in food applications, especially for maintaining the integrity of food products in moist conditions. The ability of cellulose to absorb moisture from the environment leads to significant swelling, which can affect the texture and stability of food products. This property is beneficial in certain applications such as creating moisture-retentive films or coatings for fresh produce, but it can also pose issues for dry or moisture-sensitive products [14,15].
Toxicity is another critical consideration for the use of cellulose in the food industry. While cellulose is generally regarded as safe and non-toxic, the nanoscale dimensions of cellulose particles can raise potential toxicity concerns. Factors such as particle size, surface modification, and chemical functionality influence their safety and suitability for food applications. Ensuring the safety of cellulose derivatives used in food products requires careful evaluation and adherence to regulatory standards to prevent adverse health effects [4].
The Multifaceted importance and industrial potential of cellulose
Cellulose is the most abundant organic compound on Earth, predominantly produced by plants, and constitutes the main structural component in their cells and tissues. It plays several vital roles in plant cell walls such as providing mechanical strength, supporting plant growth, aiding in cell differentiation, facilitating intercellular communication, managing water transfer, and acting as a defense mechanism. The primary cell wall is flexible and hydrated, essential for cell growth, while the secondary cell wall is more rigid, composed of cellulose, lignin, xylan, and glucomannan, enhancing structural strength and rigidity through densely packed cellulose microfibrils. To harness cellulose industrially, it is extracted via chemical processes involving agents like carbon disulfide and sulfuric acid, producing materials such as cellophane, widely used in pharmaceutical packaging for its transparency and elasticity. Cellulose versatility extends to numerous industries, including textiles, paper, and construction, and as a renewable and biocompatible energy source. It is the cornerstone of various cellulose derivatives such as cellulose ethers and esters, which are used for their stability and diverse applications in food packaging, pharmaceuticals, and other formulations [16,17].
Cellulose sources include wood, non-wood plants, marine organisms, and bacteria, each offering distinct advantages. Wood, classified into hardwood and softwood, is the primary source, with varying cellulose content affecting extraction and quality. Non-wood sources, like cotton, bamboo, and agricultural residues, are valued for their lower lignin content and environmental benefits, requiring less energy for cellulose extraction. Marine organisms such as tunicates provide high-purity cellulose, known as tunicin, which has a unique braided structure, while algae and bacteria like Acetobacter offer cellulose with high crystallinity and fewer impurities, making them ideal for specific high-value applications. The extraction process involves removing lignin and hemicellulose through methods like alkaline extraction and bleaching, which vary in complexity depending on the source material. Plant-based cellulose typically requires extensive processing, whereas marine and bacterial cellulose often contains fewer impurities, simplifying extraction and purification [49].
Behavior of cellulosic compounds in food systems
A wide variety of cellulose derivatives have been developed for use in the food industry due to their non-toxic nature. These derivatives include cellulose acetate, nitrate, and sulfate esters, as well as various cellulose ethers such as MC, HPMC, CMC, EC, HEC, HPC, and sodium carboxymethyl cellulose (NaCMC). Each of these derivatives is extensively utilized in food applications due to their unique properties and ability to enhance food quality. Cellulose derivatives like MC, HPMC, CMC, and HPC are particularly valued for their versatility in food systems. They can form gels, stabilize emulsions, and act as thickeners, binders, and film formers, which are essential for maintaining the texture, stability, and shelf life of food products. These properties make them indispensable in ensuring the quality and consistency of food items throughout processing and storage [49].
The molecular structure and size of cellulose derivatives play a crucial role in determining their functional behavior in food systems. For instance, water-soluble polymers such as CMC are ideal for applications requiring stability in aqueous environments. In contrast, water-insoluble derivatives like EC are used for formulations that need stability in non-aqueous phases. These properties are essential for producing a wide range of functional food products, including emulsifiers, stabilizers, and thickeners, which enhance the overall quality and functionality of food products. When choosing a cellulose derivative for a specific food application, several factors must be considered, including cost-effectiveness, scalability, and the specific functional requirements of the product. Often, a combination of polymers is used to achieve the desired properties, with one polymer compensating for the limitations of another. This approach allows for the development of more robust and effective formulations, tailored to meet the specific needs of different food products. The food industry continually seeks to innovate and improve the quality and stability of food products through the development of new stabilizing formulations. Companies that supply industrial raw materials are increasingly focusing on introducing innovative stabilizers that meet the evolving demands of the food sector. This has turned the design and production of food stabilizers into a profitable industry, driving advancements in food technology and ensuring the consistent quality of food products [46].
Application of cellulose derivatives in the food industry
Cellulose and its derivatives have a wide range of applications in the food industry, serving as essential processing aids and functional ingredients (Figure 6). In juice production, cellulose acts as a processing aid, facilitating the filtration process to yield clear, high-quality juices. As an anti-caking agent, it prevents clumping in powdered food products, ensuring smooth texture and easy handling. Cellulose is also a crucial component in the cheese-making process, where it is used to maintain moisture and improve texture. Furthermore, it is employed as a removable casing for hot dogs, simplifying production and enhancing product quality [46]. Different derivatives of cellulose, approved by the Food and Drug Administration (FDA), are used for various applications in the food industry. These include functioning as fat substitutes and bulking agents in low-calorie foods, which help to reduce calorie content without compromising texture or taste. Cellulose derivatives also act as emulsifiers, texturizers, and extenders, enhancing the stability and consistency of food products. They enable the production of low-calorie and low-cholesterol foods by providing the necessary bulk and texture in the absence of traditional fats and carbohydrates [46].
In addition to these applications, cellulose derivatives are utilized as fillers to reinforce hydrogels, which are used in various food formulations to decrease stickiness and improve the texture of paste-like foods. They are added to processed foods to enhance stability across a wide range of temperatures, thus improving product quality and extending shelf life. Cellulose is also integral in food packaging, where it helps maintain the safety and freshness of products over longer storage periods [46]. Various plant sources are employed for extracting cellulose, each with its unique advantages and limitations. For instance, bamboo undergoes a mercerization process that enhances the strength and stiffness of the cellulose fibers, although mechanical grinding reduces the surface area. Areca fibers provide an inexpensive and environmentally friendly source of cellulose, but the process is sensitive to temperature. Sisal fibers offer an eco-friendly extraction method that produces high-quality cellulose, though the resulting product decomposes at lower temperatures than commercial cellulose. Abaca pseudostem, treated at high temperatures, effectively removes lignin and hemicellulose, producing cellulose suitable for textile applications [46]. Diss plant fibers, which yield high-quality cellulose, have lower cellulosic content, making them suitable for producing biodegradable composites. To leaf petiole produces monoclinic cellulose I, though the crystal size may vary based on extraction and treatment methods. This cellulose is used in bio-plastic polymers and paper production. Jute fibers improve mechanical interlocking on their surface, making them ideal for biomedical composites, although they are less suitable for obtaining nanocrystals. Cellulose and its derivatives play a pivotal role in enhancing the quality, stability, and safety of food products while supporting innovations in food processing and packaging [48].[46].
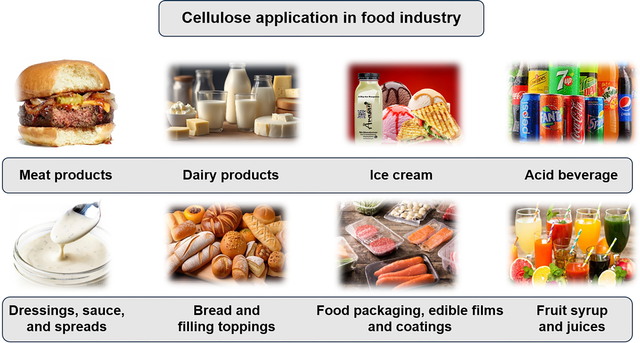
Today, cellulose and its derivatives are extensively used in the food industry. Some examples of their recent applications in food products are listed below:
MC is widely used in the food industry, particularly in plant-based meat alternatives like textured soybean burgers. It acts as a binding agent, helping to mimic the texture and sensory characteristics of meat by providing structure and moisture retention. However, its use at high concentrations has led to some consumer rejection due to preferences for healthier and more sustainable ingredients. The study explored reducing methylcellulose in soybean burgers by incorporating fructo-oligosaccharides (FOS), finding that while higher FOS concentrations resulted in softer textures and lower water holding capacity, a balanced formulation with reduced methylcellulose maintained desirable textural and sensory properties [50].
In a study, novel films were created from Hydroxypropyl methylcellulose (HPM) and cornsilk polysaccharides (CSP). Replacing 37.5% of HPM with CSP significantly enhanced the mechanical properties of the films, increasing tensile strength by 58.71% and elongation at break by 54.29%. The films also exhibited improved opacity, antioxidant activity, and a smooth surface, although thermal stability was reduced. Optimal concentrations of HPM and CSP were used to create food packaging for low-moisture mozzarella cheese, maintaining microbial stability and quality for up to 40 days of cold storage (Figure 7) [51].
CMC can be effectively used to create nano-emulsified coatings that enhance the shelf life and quality of fruits like kiwifruit. The CMC-based nano-emulsions, combined with essential oils, form a protective barrier that reduces moisture loss, microbial growth, and decay, thereby extending the freshness and marketability of the fruits (Figure 8). This application demonstrates CMC's role in maintaining food quality and safety in the food industry [52].
In the food industry, CCS is used as an effective disintegrant in the production of tablets and food supplements. For instance, in the preparation of black pepper extract tablets, CCS facilitates rapid disintegration, ensuring that the active ingredients are quickly available for absorption. This makes CCS essential for the production of fast-acting dietary supplements that maintain their effectiveness and ensure consumer satisfaction [53].
EC is used in the food industry to create intelligent packaging films. For example, an EC-based nanofilm combined with gelatin and anthocyanins from purple sweet potato can actively monitor and maintain the freshness of meat products like pork. The film responds to changes in pH and volatile compounds, providing a visual indication of spoilage through color changes, from light pink (fresh) to brownish green (spoiled) (Figure 9). This application showcases EC's potential in developing multifunctional packaging that ensures food safety and prolongs shelf life [54].
In the food industry, HEC is used to create biodegradable and edible films for packaging dairy products like sour cream. These HEC-based films, when combined with natural extracts such as olive leaf extract, provide excellent antimicrobial and antioxidant properties, extending the shelf life of the product and maintaining its quality without affecting its chemical composition. This application highlights HEC's potential in developing eco-friendly packaging solutions that enhance food safety and shelf life [55].
In the food industry, HPC is used to create biodegradable and eco-friendly packaging films. For instance, HPC can be combined with natural resins like shellac and functional nanoparticles such as TiO2 to produce packaging films with enhanced mechanical strength and antimicrobial properties. These films can be used to package perishable food items, providing a barrier against moisture and microbial contamination, thus extending the shelf life of the products while ensuring safety and sustainability [56].
In the food industry, MCC is used to create biodegradable packaging materials that help extend the shelf life of fresh produce. For example, a starch-based film with 4% MCC can be used to wrap ripe tomatoes. This film reduces water absorption and enhances biodegradability, maintaining the freshness, color, and firmness of the tomatoes for up to 12 days. The MCC in the film helps slow down decay, reduce weight loss, and preserve the fruit's nutritional content, making it an excellent choice for sustainable food packaging that protects and preserves fresh produce (Figure 10) [57].
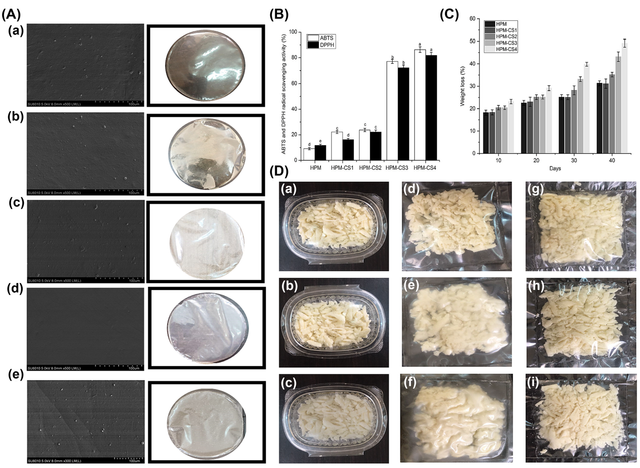
Safety assessment
The safety assessment of cellulose and its derivatives within the food industry is comprehensive and guarantees their utilization [58]. Cellulose as a normal polysaccharide obtained from plant cells and its derivatives is broadly assessed for its safety by different administrative specialists around the world with no proof of carcinogenic and mutagenic impacts. For instance, CMC has been subjected to experiments such as long-term feeding studies in animals shown no adverse effects on growth, reproduction, or organ health in various animal models which have confirmed its safety [59]. In addition to their non-toxicity, cellulose derivatives exhibit excellent stability therefore, they do not react with other food components or degrade into harmful substances during food processing and storage. Cellulose derivative’s non-digestibility by human enzymes ensures that they pass through the gastrointestinal tract unchanged which minimizes the risk of metabolic disturbances or interactions with other nutrients [60]. Likewise, cellulose and its derivatives are hypoallergenic with minimal risk of allergic reactions or sensitivities due to the absence of protein in these compounds which reduces the potential for allergenicity and makes them suitable for a wide range of consumers involving those with dietary limitations [61]. This is particularly important in the food industry where the frequency of food allergies and intolerances is an upward concern. Overall, the robust safety outline of cellulose and its derivatives in the food industry has been supported by extensive scientific research and regulatory approvals.
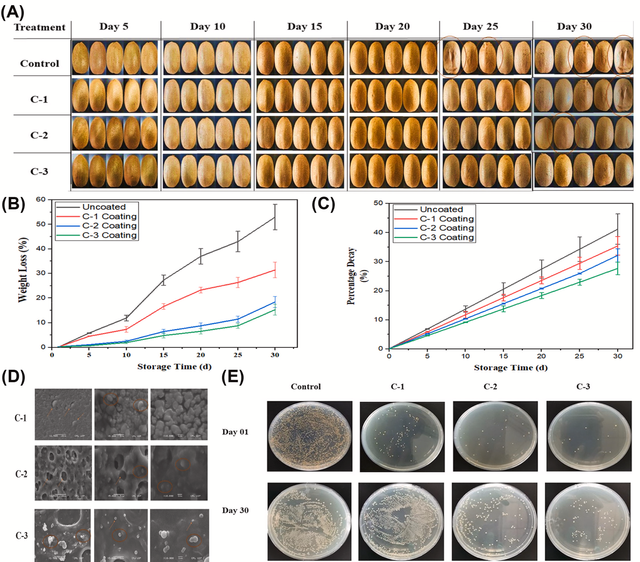
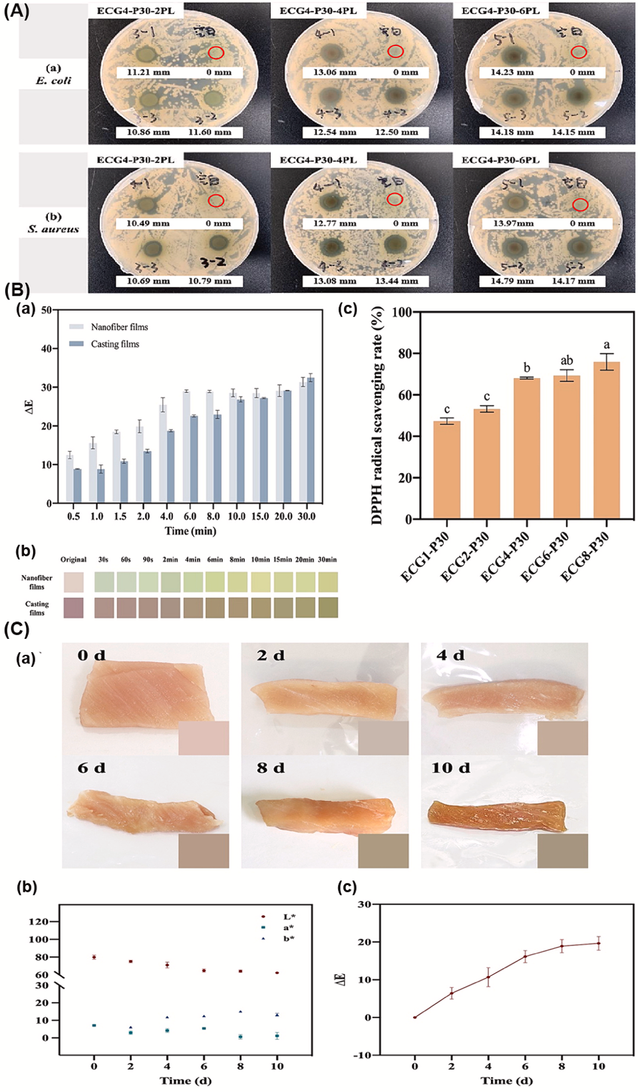
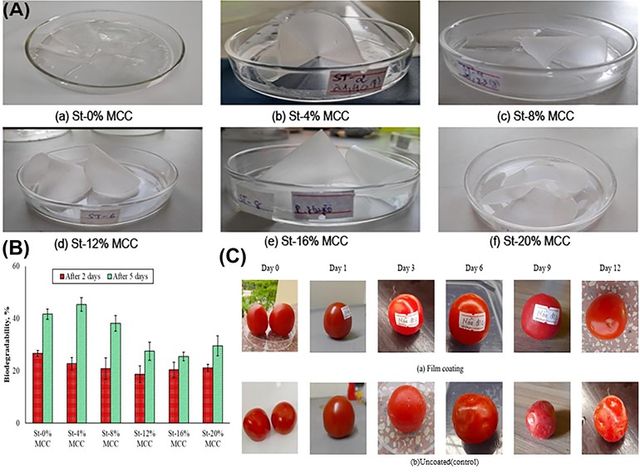
Conclusion and perspective
The significant findings of this work highlight the crucial role of cellulose and its derivatives in enhancing food quality, texture, and shelf life. Their multifunctional properties as thickeners, stabilizers, emulsifiers, and gelling agents have proven indispensable in the development of a wide range of food products from bakery and dairy items to sauces and processed foods. Additionally, their non-toxic, hypoallergenic, and biodegradable nature aligns perfectly with consumer demands for safe, healthy, and sustainable food ingredients. Looking forward, the potential of cellulose derivatives to meet the growing demand for functional foods that promote health is promising, particularly due to their dietary fiber content and versatile applications. Advances in nanotechnology and materials science open up new avenues for the development of cellulose nanocomposites with customized properties for specific food applications. The integration of smart technologies such as sensors and indicators within these nanocomposites could revolutionize food packaging by providing real-time monitoring of food quality and safety.
Innovations like MCC and CMC enable precise control over food properties that open up new possibilities for product development and food preservation. In conclusion, ongoing research and development are composed to further enhance the role of cellulose biomaterials contributing to a more sustainable, efficient, and safe food industry.
The future of cellulose and its derivatives in the food industry looks promising, driven by the demand for sustainable, health-conscious, and innovative products. As a natural and renewable ingredient, cellulose is increasingly valued for its versatility in enhancing texture, stability, and nutritional content. In plant-based and functional foods, derivatives like methylcellulose are key to mimicking meat textures and improving product appeal. Additionally, cellulose's role as a dietary fiber is gaining adhesion that offers benefits for gut health and enables the development of healthier, low-calorie options. The trend towards clean-label products further enhances the use of cellulose as it can replace synthetic additives with a natural alternative. As consumer preferences evolve, cellulose is set to play a crucial role in the future of food innovation supporting the creation of sustainable and health-focused products.
Statements and Declarations
Authors' contributions
N. Biglari and A. Serajian contributed to the conception of the study. N. Biglari and A. Serajian wrote the first and final drafts of the manuscript.
Competing interests
The author declares no competing interest.
Ethics approval
Not applicable.
Data availability
Not applicable.
Funding
This paper received no external funding.
Authors’ Information
Nazila Biglari—School of Biological Sciences, Universiti Sains Malaysia, Penang, Malaysia;
Azam Serajian—Department of Chemistry, Institute for Advanced Studies in Basic Sciences (IASBS), Zanjan, Iran.