Visual Abstract
Abstract
ZnO nanorods with hexagonal structures were synthesized using a hydrothermal method under different conditions. The effect of synthesis parameters on ZnO growth was systematically studied by scanning electron microscopy. The results demonstrated that the morphology and size of ZnO nanorods are influenced by nature of the solvent, zinc acetate concentration, growth temperature and time. Rod-like and needle-shaped ZnO was produced when DI water and ethanol were used as the solvent, respectively. The concentration of precursors can also influence the formation of ZnO nanorods. Increasing growth time leads to rod-like diameter increment. ZnO nanorods with desired diameters were successfully prepared using 2.3 M zinc acetate concentration in DI water with a growing temperature of 80 ºC for 3 h. Next, Au/ZnO nanorod heterostructure was synthesized by a self-assembly of Au salt precursors and subsequent reduction of Au ions on ZnO nanorod surface without any surfactants or additives. Through SEM analysis, it was found that Au nanoparticles were uniformly deposited on the surface of ZnO nanorods. Compared with bare ZnO nanorods, Au/ZnO heterostructures exhibited characteristic surface plasmon absorption at 900 nm.
Introduction
In recent years, tremendous attention has been drawn toward using one-dimensional (1D) zinc oxide (ZnO) nanomaterial in diverse applications owing to their superb properties such as optical, semiconducting, chemical sensing, electric conductivity, piezoelectric and antibacterial features [1]. It is well-known for its high excitonic binding energy (60 meV) at ambient temperature and a direct wide band gap (3.3 eV) in the near-UV spectrum [2]. These features enable ZnO nanorods to have remarkable applications in different fields such as biosensors [3–4], photocatalysts [5], tissue engineering [6–7], and cancer therapy [8].
The concept of green biomaterials emphasizes the development and utilization of sustainable materials that minimize environmental impact while offering innovative solutions for various applications in research, education, industry, and clinical settings [9]. In the context of advancing green biomaterials principles, several key points can be discussed regarding the use of materials like ZnO nanorods and Au nanoparticles in this context: 1) Sustainability and Ecological Footprint: ZnO nanorods and Au nanoparticles synthesized using green chemistry principles, which involve environmentally friendly method. 2) Biocompatibility and Biodegradability: Green biomaterials, including ZnO nanorods and Au nanoparticles, are designed to be biocompatible and biodegradable, ensuring minimal harm to living systems and the environment [9]. This characteristic is essential for their safe use in biomedical applications, such as drug delivery and tissue engineering. 3) Reduced Toxicity and Environmental Impact: Compared to conventional biomaterials synthesized using toxic chemicals, green biomaterials like ZnO nanorods and Au nanoparticles offer reduced toxicity and environmental impact throughout their lifecycle. This aligns with the shift towards eco-friendly practices in material science and healthcare [9].
Different synthesis methods have been utilized to synthesize ZnO nanorods such as pulse laser deposition [10], electrochemical deposition [11], and chemical vapor deposition (CVD) [12]. However, the growth temperatures of such techniques are high (> 350 ⁰C) and these methods are somehow complicated. On the other hand, the solvothermal process has many advantages such as low process temperature, better homogeneity, easier composition control, and the most economical for large-scale production of ZnO nanorods among solution-based methods [13].
Since the shape and size of the nanostructures can affect the properties of materials at the nanoscale, it is crucial to control these parameters during the synthesis process [14]. The morphology and size of ZnO can be influenced by many parameters, such as preparation method, growing temperature [15], nature of the solvent [16], pH [17], concentration of the salt, and growing time duration [18]. Although an attempt was made to understand the influence of synthesis parameters on the size and morphology of ZnO, it is still desired to know more in this regard.
The surface modification of ZnO nanostructures is an effective strategy to improve their properties [19–20]. For instance, the surface of ZnO nanostructures could be decorated by metal nanoparticles (NPs) to construct metal-ZnO nano-architectures [20–21]. In this regard, Au NPs have a high potential to deposit on the surface of ZnO nanorods for enhancing the optical, electrical as well as sensing properties due to their optical sensitivity, catalytic activity, biocompatibility, and chemical stability [19–21].
To decorate the Au NPs on ZnO nanorods, there are different gold loading methods, such as ion beam sputtering, hydrothermal process, electrophoretic deposition, and photochemical synthesis [22–24]. However, such methods need a relatively long synthesis time duration, additional reaction agents, or specific substrates to help the reduction of Au ions [22–24]. Although some progress has been achieved in the synthesis of different ZnO/Au nanostructures, much room remains for developing such structures easily and rapidly with no additive requirements. Moreover, constructing controllable surface coverage of the nanostructure is also essential.
In this study, the novelty lies in the comprehensive optimization of synthesis parameters for ZnO nanorods with the specific objective of enhancing their photocatalytic performance through the decoration of Au nanoparticles. The research delves into the systematic exploration of various factors influencing the growth of ZnO nanorods, such as the nature of the solvent, zinc acetate concentration, growth temperature, and time. By meticulously studying the impact of these parameters on the morphology and size of ZnO nanorods, the research aims to achieve a tailored design for optimal photocatalytic activity. Moreover, introducing Au/ZnO nanorod heterostructures through a surfactant-free self-assembly process represents a unique aspect of this work. The successful deposition of Au nanoparticles onto the surface of ZnO nanorods without additional additives or surfactants showcases the innovative approach adopted in this research.
Materials and Methods
Materials
Zinc acetate dehydrate (Zn(CH3CO2)2.2H2O), sodium hydroxide (NaOH, ≥97%, pellets form), ethylenediamine (EDA, C2H8N2), ethanol 96%, hydrogen tetrachloroaurate (III) trihydrate (HAuCl4.3H2O, 99.5% purity), sodium citrate dihydrate (C6H5Na3O7.2H2O) were purchased from Merck, Germany. All the reagents used in this research were of analytical grade and used without further purification.
Synthesis of zinc oxide nanorods
ZnO nanorods were synthesized using the hydrothermal method. For this purpose, an appropriate amount of Zn(CH3CO2)2.2H2O (0.6 M, 1.2 M, and 2.3 M) was dissolved in deionized water. Aqueous solution of NaOH (2.1 g in 2 ml of deionized water) was added slowly into the resulting solution. The prepared mixture was kept under constant stirring for 2 h. After this period, 50 ml of solvent (ethanol or deionized water) and 2.5 ml of EDA were added to the mixture and stirred in a plastic flask for 30 minutes at 60 ºC. Then, the mixture was moved to an autoclave and kept at a certain temperature (60 ºC, 80 ºC, and 120 ºC) for different duration of time (1.5 h, 2 h, 3 h, 4 h).
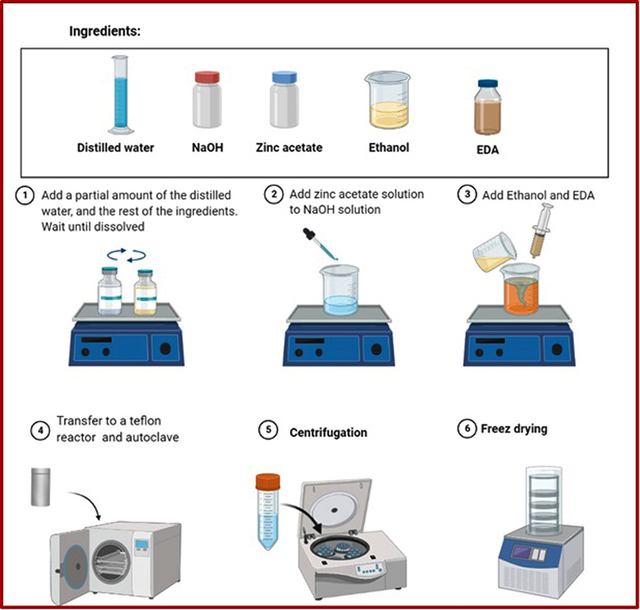
Finally, the solution was centrifuged and washed with deionized water and ethanol before freezer drying for 24 h. The schematic illustration of the synthesis of ZnO nanorods is shown in Figure 1.
Decorating of Au nanoparticles on ZnO nanorods
The decorating of AuNPs on ZnO NRs surface was carried out via applying HAuCl4.3H2O as a precursor with some modification. Briefly, the as-prepared ZnO NRs (50 mg) were dispersed to (40 mL) deionized H2O. Then, 0.05 ml of HAuCl4.3H2O solution with a certain concentration of 50 mM was added to the stirring ZnO solution. Afterward, 2 ml NH3.H2O was added to the above solution. The solution was stirred for 2 h at room temperature for 5 min. 2 h later, the as-synthesized purple solid product was collected by centrifugation, washed with H2O several times, and dried at 70 °C in an air atmosphere for 5 h.
Characterization techniques
The composition, morphology, and size of ZnO nanostructures as well as Au/ZnO nanocomposite were studied by FESEM using TESCAN VEGA3 at a 5 kV accelerating voltage. The crystalline structure of the samples was examined by an X-ray diffractometer (Philips, PW1730, 40 kV, 30 mA). Briefly, 200 µl of the products were dispersed in water, dropped on a silicon substrate, and finally dried at ambient temperature. The optical properties of the samples as well as the formation of AuNPs on ZnO nanorods were investigated by UV–visible (UV–Vis) absorption spectroscopy (Shimadzu UV–160A).
Results and discussion
Synthesis of 1D ZnO nanomaterial
Solvent effect on ZnO nanorods growth
Physicochemical features of the solvent, as the reaction medium for ZnO synthesis, have a significant effect on both the formation of ZnO nuclei and the growth of the crystals [25]. Figure 2 shows FESEM images of the grown ZnO nanostructures in two different solvents, DI water and ethanol 96%. As shown in Figure 2, a very slender needle-like ZnO was obtained using ethanol as a solvent. However, rod-like morphology with a hexagonal shape in the cross-section was achieved using DI water as a solvent. This was assigned to the growth rates of specific planes of the ZnO nanorod [17]. A similar rod-like morphology was reported by Zhang et al. in which growth occurred at higher temperatures [26]. The diameter and length of the ZnO nanorod synthesized in DI water were found to be 253±61 nm and 1.23±0.34 µm, respectively (Figure 2c). Ethylenediamine is a famous chelating ligand for coordination compounds, with the two nitrogen atoms donating their lone pairs of electrons when ethylenediamine acts as a ligand. Researchers show that zinc with ethylene diamine forms a [Zn(en)2]2+ complex. As the temperature increases, the stability of the complex decreases. Under these conditions, oxygen atoms are reduced (O changes to O–2). Anions attack the complex resulting from solvent and zinc, breaking the bonds of zinc with solvent nitrogen atoms. So a weak bond is formed between zinc ions and oxygen, eventually creating a one-dimensional ZnO structure.
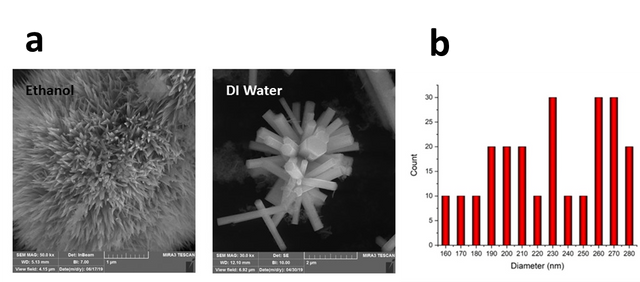
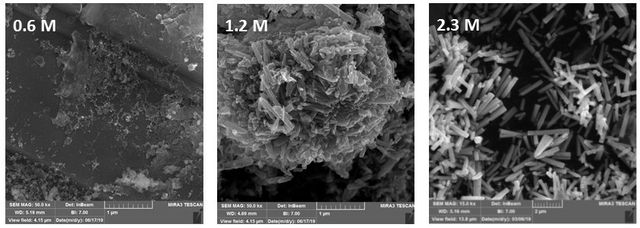
Precursor concentration effect on ZnO nanorods growth
To study the influence of precursor concentration on the shape of ZnO samples, various amounts of zinc acetate in DI water (0.6 M, 1.2 M, and 2.3 M) were prepared at constant growing temperature and time of 80 ºC and 3 h, respectively. As can be seen in Figure 3, at low precursor concentrations, ZnO nanorods did not form.
Temperature effect on ZnO nanorods growth
Figure 4. displays SEM images of samples grown at 60, 80, and 120 ⁰C. When the synthesis process was performed at a lower temperature (60 ⁰C), the ZnO nanorods could hardly be seen in the SEM images. It seems that this temperature was not sufficient for ZnO nanorod’s growth. Figure 4 reveals the morphology of nanorods grown at 80 ⁰C under the same conditions. These nanorods show a diameter of 55 nm and a length of 2 µm. Meanwhile, by increasing the growing temperature to 120 ⁰C, thick ZnO with rod-like morphology was obtained and the uniformity of the rods decreased. The possible reason for this shape transformation was the increased kinetic energy of the nanorods molecules at high temperatures. Therefore, experimental data show that the temperature of the reactor plays a key role in the formation of the ZnO nano/micro rods.
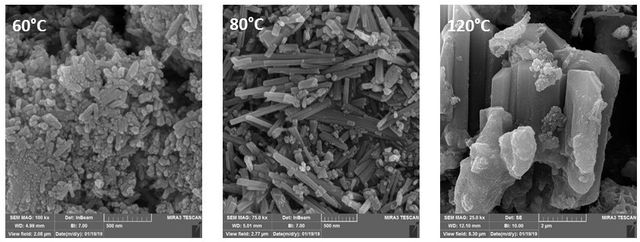
Incubation time effect on ZnO nanorod’s growth
The dependence of the average length and diameter of nanorods on incubation time is shown in Figure 5. As can be seen, the formation rate of the nanorods is very low at 1.5 h and 2 h. Since the supersaturation of the solution is high, the main process in this initial stage might be the formation of hexagonal ZnO nanocrystalline grains on which the nanorods grow. By increasing the incubation time, the formation of nanorods boosts and the increment of nanorods density can be distinguishable (Figure 6, 3h and 4h). Kinetic studies have shown that the growth of ZnO nanorods happens as follows: (i) fast steps within the first hour, in which the nanorods tend to be short and wide, and (ii) a slow step, in which long rods with high aspect ratio are formed. In other words, the growth in the radial direction occurs in the early stage of the hydrothermal process (within the first 2 h), and consequently, ZnO nanorods with a small aspect ratio tend to be formed in this stage. Then, the radial growth rate diminishes while the axial growth rate remains at a relatively high level, resulting in long rods with a high aspect ratio [27].
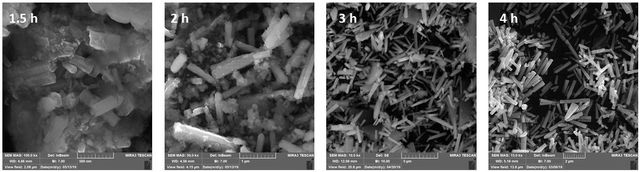
The dependence of the average diameter and length of nanorods on deposition time is shown in Table 1. It has also been reported that the growth time can affect the diameter and length of the ZnO crystallites. Based on the results of FESEM images as well as the size distribution histogram, the optimum incubation time was considered to be 3 h.
Incubation time (h) | Diameter of ZnO NRs (nm) | Length of ZnO NRs (nm) | Aspect ratio |
1.5 | 265±53 | 319±68 | 1.2 |
2 | 280±42 | 818±75 | 2.9 |
3 | 314±33 | 1239±54 | 3.9 |
4 | ±38 | 1391±62 | 4.0 |
Structural characterization
Figure 6 shows the XRD pattern of ZnO synthesized in DI water. A series of characteristic peaks: 31.37 ⁰ (100), 34.38 ⁰ (002), 36.21⁰ (101), 47.48⁰ (102), 56.54⁰ (110), 62.77⁰ (103), 66.31⁰ (200), 67.87⁰ (112) and 69.01⁰ (203) were observed and they were by the typical wurtzite hexagonal structure of ZnO in the reference data (JCPDS No. 36–1451). The sharp and narrow diffraction peaks indicate that the material has good crystallinity. No characteristic peaks from the intermediates such as Zn(OH)2 can be detected.
The positions of diffraction peaks can provide information about the crystallographic orientation and quality of the ZnO nanorods. (101) peak has the highest corrected intensity (Ic), indicating a strong diffraction signal from the (101) crystallographic plane. This suggests a potential preferred orientation along the (101) direction.
The degree of c-orientation can be determined by the relative texture coefficient, which was calculated using the following expression [17].
\(\text{TC}(hkl)=\frac{I(hkl)/I_0(hkl)}{\frac{1}{N}\sum\limits_{N}\frac{I(hkl)}{I_0(hkl)}}\)
As can be seen in Table 2, \(\text{TC}(hkl)\) of 1 represents a sample with randomly oriented crystallites while a larger value indicates an abundance of crystallites oriented to the \((hkl)\) plane.
2θ (º) | (hkl) | I0 | I | TC |
31.37 | 100 | 57 | 248 | 0.23 |
34.38 | 002 | 44 | 302 | 0.37 |
36.21 | 101 | 100 | 899 | 0.48 |
47.48 | 102 | 23 | 352 | 0.64 |
56.54 | 110 | 32 | 468 | 0.82 |
62.77 | 103 | 29 | 587 | 1.08 |
66.31 | 200 | 4 | 222 | 2.97 |
67.87 | 112 | 23 | 534 | 1.24 |
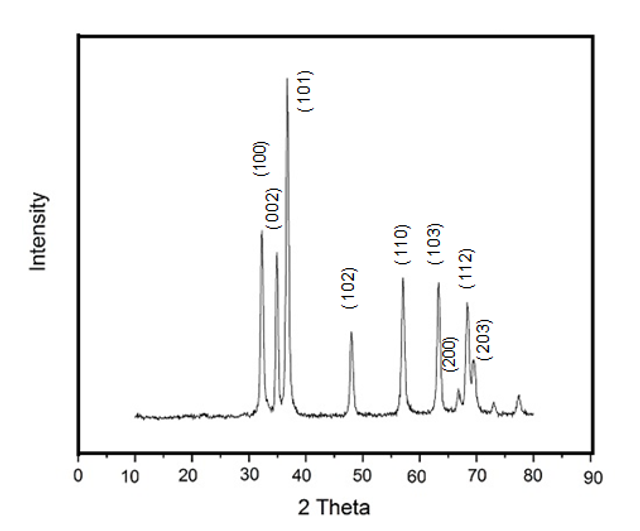
Decorating of gold nanoparticles on ZnO nanorod’s surface
Noble metal nanoparticles with different morphologies and sizes have great interest due to their unique electronic and optical properties that originate from their localized surface plasmon resonance [28]. Among the noble metal nanoparticles, the combination of gold nanoparticles with ZnO nanorods can play an essential role in different fields such as cancer diagnosis and biological imaging, photocatalysis/photodetector, energy storage, chemical sensors, and environmental restoration.
In the current work, Au nanoparticles -decorated ZnO nanorod heterostructure was fabricated under appropriate growth conditions. For this purpose, first, the ZnO nanorods were synthesized according to the optimized condition obtained from the previous section (2.3 M zinc acetate solution in water, growth temperature: 80 ⁰C, and incubation time: 3 h). When ZnO nanorods are decorated with Au nanoparticles, a hetero-nanostructure with a rough surface is obtained. The FESEM image in Figure 7. shows that each Au NP was closely anchored to a ZnO nanorod and no support-free particles were detected in the Au-loaded ZnO nanostructure. These nearly spherical Au NPs with average sizes of approximately 12 nm were highly dispersed along ZnO nanorods in high coverage, providing high-level surface exposure of Au NPs. The decorating of AuNPs on the ZnO nanorod surfaces can be explained by as follows: In the nucleation step, the dispersed ZnO nanorods act as seed materials for Au nucleation. For the growth of AuNPs, the Au ions in the solvent are adsorbed on the ZnO nanorod surface where the electrons are accumulated and reduced to Au0 to grow as nanoparticles. In other words, the Au seed acts as an electron storage [28].
The Au/ZnO nanocomposites were studied by energy-dispersive (EDX) test. The X-ray spectrum (EDX) (Figure 7.) determined from the purified nanoproduct shows the peaks related to Au, Zn, and O elements presented in the final nanoproduct Au/ZnO. The weight percentage of Au in the ZnO/Au heterostructure was found to be 24.8%.
Figure 8. shows the UV-vis absorption spectra of pure ZnO nanorods and ZnO/Au nanocomposites. The sharp absorption peak at about 370 nm corresponded to a well-defined exciton band of pure ZnO nanorods. The absorption of ZnO/Au nanocomposite is similar to that of ZnO particle apart from the broad absorption band centered at about 900 nm which was ascribed to the surface plasmon resonance of AuNPs.
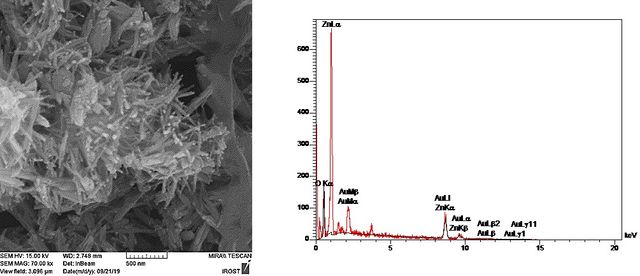
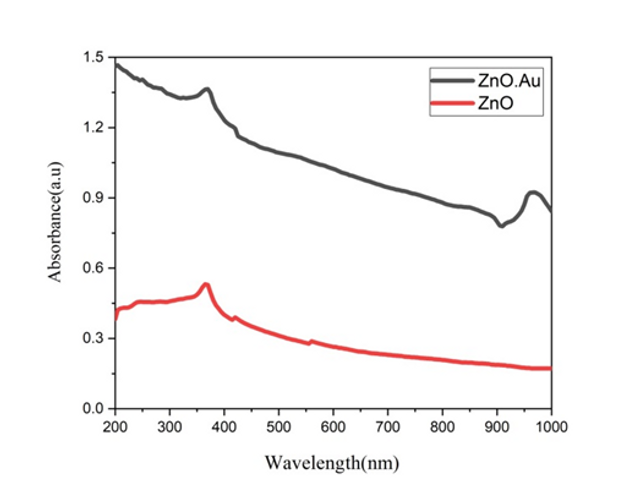
Conclusion
In summary, the nature of the solvent, the concentration of the precursors, the growing temperature, and incubation time have a great influence on the morphology of ZnO nanorods. Using water as a solvent led to rod-like ZnO while needle-like ZnO was obtained in ethanol. The concentration of the precursors is crucial for the formation of ZnO. 80 ⁰C and 3 h were considered as the optimum growth temperature and time for rod-like ZnO formation, respectively. The results can guide a better understanding of the growth behavior of ZnO nanorods as a function of synthesis parameters and can contribute to the development of novel nanodevices.
Moreover, Au nanoparticles deposited on ZnO nanorods hetero-nanostructure have been synthesized via self-assembly of Au salt precursors and subsequent reduction of Au ions on the ZnO nanorod surface. It was confirmed that several nanometer-sized Au nanoparticles were uniformly deposited on the ZnO nanorod surface using SEM analysis. The ZnO/Au nanohybrids show characteristic surface plasmon absorption at 370 nm.
Statements and Declarations
Authors' contributions
Atena Aliakbar Shirazi: Methodology, Investigation, Analysis, and interpretation of data and software; Najmeh Najmoddin: Supervision, Conceptualization, Project Administration, Analysis and interpretation of data, Writing-Original draft; Neda Attaran: Supervision, Conceptualization, Analysis, and interpretation of data, Resources, Writing-Review and Editing.
Competing interests
The authors declare no competing interests.
Ethics approval
Not applicable.
Data availability
All data generated or analyzed during this study are included in this published article.
Funding
No funding was received to support this work.
Authors’ Information
Atena Aliakbar Shirazi—Department of Biomedical Engineering, Medical Engineering and Biology Research Center, Science and Research Branch, Islamic Azad University, Tehran, Iran.
Najmeh Najmoddin—Department of Biomedical Engineering, Medical Engineering and Biology Research Center, Science and Research Branch, Islamic Azad University, Tehran, Iran;
orcid.org/0000-0002-1085-665X
Neda Attaran—Department of Medical Nanotechnology, Applied Biophotonics Research Center, Science and Research Branch, Islamic Azad University, Tehran, Iran;